人多能干细胞在环境污染物风险评估中的应用与展望
Application and Prospect of Human Pluripotent Stem Cells in Risk Assessment of Environmental Pollutants
-
摘要: 在环境污染问题日益严峻的今天,人们亟需一套高效的毒理学评价体系来全面评估各类环境污染物的毒性效应和致毒机制,并阐明化合物结构与毒性效应之间的关系,进而指导安全化合物的合成。人多能干细胞(hPSCs)具有近乎无限的增殖能力和分化成所有成体细胞的潜能,近年来在毒理学的应用中崭露头角,显现出极大的应用潜力。由hPSCs分化而来的细胞可以代替原代细胞进行高通量的毒理学研究;hPSCs分化模型便于在体外研究环境污染物暴露对人体胚胎发育过程的毒性;基于hPSCs构建的类器官技术也使环境污染物的器官毒性研究成为可能。hPSCs在环境污染物风险评估中有很高的应用价值。Abstract: With environmental issues being increasingly serious, an efficient toxicology evaluation system is urgently needed to comprehensively evaluate the toxic effects of various environmental pollutants, reveal the toxic mechanism, discern the relationship between the structure and toxic effects of chemicals, and thus guide the synthesis of safe compounds. In recent years, the application of human pluripotent stem cells (hPSCs) in toxicity research has emerged showing great potential. hPSCs possess almost unlimited proliferation ability and the potential to differentiate into all the cell types of the adult. Cells differentiated from hPSCs can replace primary cells for toxicity research and the experiments can be carried out in a high-throughput manner. The differentiation model of hPSCs can be used to study the developmental toxicity of environmental pollutants to human embryos in vitro. In addition, technology breakthroughs of iPSC-based organoid construction make it possible to study the organ toxicity of environmental pollutants. Therefore, hPSCs possess great practical value in risk assessment of environmental pollutants.
-
-
Zhao X Y, Li W, Lv Z, et al. iPS cells produce viable mice through tetraploid complementation[J]. Nature, 2009, 461(7260):86-90 Evans M J, Kaufman M H. Establishment in culture of pluripotential cells from mouse embryos[J]. Nature, 1981, 292(5819):154-156 Laschinski G, Vogel R, Spielmann H. Cytotoxicity test using blastocyst-derived euploid embryonal stem cells:A new approach to in vitro teratogenesis screening[J]. Reproductive Toxicology, 1991, 5(1):57-64 Spielmann H, Pohl I, Doring B, et al. The embryonic stem cell test (EST), an in vitro embryotoxicity test using two permanent mouse cell lines:3T3 fibroblasts and embryonic stem cells[J]. Toxicology in Vitro, 1997, 10:119-127 Thomson J A, Itskovitz-Eldor J, Shapiro S S, et al. Embryonic stem cell lines derived from human blastocysts[J]. Science, 1998, 282(5391):1145-1147 Scholz G, Pohl I, Genschow E, et al. Embryotoxicity screening using embryonic stem cells in vitro:Correlation to in vivo teratogenicity[J]. Cells Tissues Organs, 1999, 165(3-4):203-211 Takahashi K, Tanabe K, Ohnuki M, et al. Induction of pluripotent stem cells from adult human fibroblasts by defined factors[J]. Cell, 2007, 131(5):861-872 Park I H, Arora N, Huo H, et al. Disease-specific induced pluripotent stem cells[J]. Cell, 2008, 134(5):877-886 Adler S, Pellizzer C, Hareng L, et al. First steps in establishing a developmental toxicity test method based on human embryonic stem cells[J]. Toxicology in Vitro, 2008, 22(1):200-211 Guo L, Abrams R M, Babiarz J E, et al. Estimating the risk of drug-induced proarrhythmia using human induced pluripotent stem cell-derived cardiomyocytes[J]. Toxicological Sciences, 2011, 123(1):281-289 Rowe R G, Daley G Q. Induced pluripotent stem cells in disease modelling and drug discovery[J]. Nature Reviews Genetics, 2019, 20(7):377-388 Pei Y, Peng J, Behl M, et al. Comparative neurotoxicity screening in human iPSC-derived neural stem cells, neurons and astrocytes[J]. Brain Research, 2016, 1638(Pt A):57-73 Rice D, Barone S Jr. Critical periods of vulnerability for the developing nervous system:Evidence from humans and animal models[J]. Environmental Health Perspectives, 2000, 108(Suppl 3):511-533 Kadereit S, Zimmer B, van Thriel C, et al. Compound selection for in vitro modeling of developmental neurotoxicity[J]. Frontiers in Bioscience (Landmark Ed.), 2012, 17:2442-2460 Colleoni S, Galli C, Gaspar J A, et al. Development of a neural teratogenicity test based on human embryonic stem cells:Response to retinoic acid exposure[J]. Toxicological Sciences, 2011, 124(2):370-377 Hoelting L, Scheinhardt B, Bondarenko O, et al. A 3-dimensional human embryonic stem cell (hESC)-derived model to detect developmental neurotoxicity of nanoparticles[J]. Archive of Toxicology, 2013, 87(4):721-733 Huang B, Ning S, Zhang Q, et al. Bisphenol A represses dopaminergic neuron differentiation from human embryonic stem cells through downregulating the expression of insulin-like growth factor 1[J]. Molecular Neurobiology, 2017, 54(5):3798-3812 Krug A K, Kolde R, Gaspar J A, et al. Human embryonic stem cell-derived test systems for developmental neurotoxicity:A transcriptomics approach[J]. Archive of Toxicology, 2013, 87(1):123-143 Chen H, Seifikar H, Larocque N, et al. Using a multi-stage hESC model to characterize BDE-47 toxicity during neurogenesis[J]. Toxicological Sciences, 2019, 171(1):221-234 Trevino L S, Katz T A. Endocrine disruptors and developmental origins of nonalcoholic fatty liver disease[J]. Endocrinology, 2018, 159(1):20-31 Liang S, Liang S, Yin N, et al. Establishment of a human embryonic stem cell-based liver differentiation model for hepatotoxicity evaluations[J]. Ecotoxicology and Environmental Safety, 2019, 174:353-362 van der Linde D, Konings E E, Slager M A, et al. Birth prevalence of congenital heart disease worldwide:A systematic review and meta-analysis[J]. Journal of the American College of Cardiology 2011, 58(21):2241-2247 Hoffman J I E, Kaplan S. The incidence of congenital heart disease[J]. Journal of the American College of Cardiology, 2002, 39(12):1890-1900 Fu H, Wang L, Wang J, et al. Dioxin and AHR impairs mesoderm gene expression and cardiac differentiation in human embryonic stem cells[J]. Science of the Total Environment, 2019, 651(Pt 1):1038-1046 Sant K E, Jacobs H M, Borofski K A, et al. Embryonic exposures to perfluorooctanesulfonic acid (PFOS) disrupt pancreatic organogenesis in the zebrafish, Danio rerio[J]. Environmental Pollution, 2017, 220(Pt B):807-817 Liu S, Yin N, Faiola F. PFOA and PFOS disrupt the generation of human pancreatic progenitor cells[J]. Environmental Science & Technology Letters, 2018, 5(5):237-242 Lind L, Zethelius B, Salihovic S, et al. Circulating levels of perfluoroalkyl substances and prevalent diabetes in the elderly[J]. Diabetologia, 2014, 57(3):473-479 Karnes C, Winquist A, Steenland K. Incidence of typeⅡ diabetes in a cohort with substantial exposure to perfluorooctanoic acid[J]. Environmental Research, 2014, 128:78-83 Domazet S L, Grontved A, Timmermann A G, et al. Longitudinal associations of exposure to perfluoroalkylated substances in childhood and adolescence and indicators of adiposity and glucose metabolism 6 and 12 years later:The European Youth Heart Study[J]. Diabetes Care, 2016, 39(10):1745-1751 Conway B, Innes K E, Long D. Perfluoroalkyl substances and beta cell deficient diabetes[J]. Journal of Diabetes and Its Complications, 2016, 30(6):993-998 Cardenas A, Gold D R, Hauser R, et al. Plasma concentrations of per- and polyfluoroalkyl substances at baseline and associations with glycemic indicators and diabetes incidence among high-risk adults in the diabetes prevention program trial[J]. Environmental Health Perspectives, 2017, 125(10):107001 Gurtner G C, Werner S, Barrandon Y, et al. Wound repair and regeneration[J]. Nature, 2008, 453(7193):314-321 Culton D A, Qian Y, Li N, et al. Advances in pemphigus and its endemic pemphigus foliaceus (Fogo Selvagem) phenotype:A paradigm of human autoimmunity[J]. Journal of Autoimmunity, 2008, 31(4):311-324 Cheng Z, Liang X, Liang S, et al. A human embryonic stem cell-based in vitro model revealed that ultrafine carbon particles may cause skin inflammation and psoriasis[J]. Journal of Environmental Sciences, 2020, 87:194-204 Haycock J W. 3D cell culture:A review of current approaches and techniques[J]. Methods in Molecular Biology, 2011, 695:1-15 Lancaster M A, Knoblich J A. Organogenesis in a dish:Modeling development and disease using organoid technologies[J]. Science, 2014, 345(6194):1247125 Takebe T, Sekine K, Enomura M, et al. Vascularized and functional human liver from an iPSC-derived organ bud transplant[J]. Nature, 2013, 499(7459):481-484 Pasca S P. The rise of three-dimensional human brain cultures[J]. Nature, 2018, 553(7689):437-445 Richards D J, Coyle R C, Tan Y, et al. Inspiration from heart development:Biomimetic development of functional human cardiac organoids[J]. Biomaterials, 2017, 142:112-123 Huch M, Gehart H, van Boxtel R, et al. Long-term culture of genome-stable bipotent stem cells from adult human liver[J]. Cell, 2015, 160(1-2):299-312 Leite S B, Roosens T, El Taghdouini A, et al. Novel human hepatic organoid model enables testing of drug-induced liver fibrosis in vitro[J]. Biomaterials, 2016, 78:1-10 Boj S F, Hwang C I, Baker L A, et al. Organoid models of human and mouse ductal pancreatic cancer[J]. Cell, 2015, 160(1-2):324-338 Barkauskas C E, Chung M I, Fioret B, et al. Lung organoids:Current uses and future promise[J]. Development, 2017, 144(6):986-997 Taguchi A, Nishinakamura R. Higher-order kidney organogenesis from pluripotent stem cells[J]. Cell Stem Cell, 2017, 21(6):730-746.e6 Kessler M, Hoffmann K, Brinkmann V, et al. The Notch and Wnt pathways regulate stemness and differentiation in human fallopian tube organoids[J]. Nature Communication, 2015, 6:8989 Zhong X, Gutierrez C, Xue T, et al. Generation of three-dimensional retinal tissue with functional photoreceptors from human iPSCs[J]. Nature Communications, 2014, 5:4047 Foster J W, Wahlin K, Adams S M, et al. Cornea organoids from human induced pluripotent stem cells[J]. Scientific Reports, 2017, 7:41286 Maimets M, Rocchi C, Bron R, et al. Long-term in vitro expansion of salivary gland stem cells driven by Wnt signals[J]. Stem Cell Reports, 2016, 6(1):150-162 Turco M Y, Gardner L, Hughes J, et al. Long-term, hormone-responsive organoid cultures of human endometrium in a chemically defined medium[J].Nature Cell Biology, 2017, 19(5):568-577 Titmarsh D M, Nurcombe V, Cheung C, et al. Vascular cells and tissue constructs derived from human pluripotent stem cells for toxicological screening[J]. Stem Cells and Development, 2019, 28(20):1347-1364 Schwartz M P, Hou Z, Propson N E, et al. Human pluripotent stem cell-derived neural constructs for predicting neural toxicity[J].Proceedings of the National Academy of Sciences of the United States of America, 2015, 112(40):12516-12521 Mills R J, Parker B L, Quaife-Ryan G A, et al. Drugscreening in human PSC-cardiac organoids identifies pro-proliferative compounds acting via the mevalonate pathway[J]. Cell Stem Cell, 2019, 24(6):895-907.e6 李朋彦, 李春雨, 陆小华, 等. 基于类器官3D培养和高内涵成像的药物肝毒性评价模型研究[J]. 药学学报, 2017, 52(7):1055-1062 Li P Y, Li C Y, Lu X H, et al. The three dimensional organoids-based high content imaging model for hepatotoxicity assessment[J]. Acta Pharmaceutica Sinica, 2017, 52(7):1055-1062(in Chinese)
Czerniecki S M, Cruz N M, Harder J L, et al. High-throughput screening enhances kidney organoid differentiation from human pluripotent stem cells and enables automated multidimensional phenotyping[J]. Cell Stem Cell, 2018, 22(6):929-940.e4 Li X J, Valadez A V, Zuo P, et al. Microfluidic 3D cell culture:Potential application for tissue-based bioassays[J]. Bioanalysis, 2012, 4(12):1509-1525 van Duinen V, Trietsch S J, Joore J, et al. Microfluidic 3D cell culture:From tools to tissue models[J]. Current Opinion in Biotechnology, 2015, 35:118-126 Huh D, Matthews B D, Mammoto A, et al. Reconstituting organ-level lung functions on a chip[J]. Science, 2010, 328(5986):1662-1668 Park S E, Georgescu A, Huh D. Organoids-on-a-chip[J]. Science, 2019, 364(6444):960-965 -
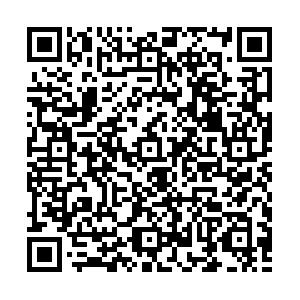
计量
- 文章访问数: 2561
- HTML全文浏览数: 2561
- PDF下载数: 92
- 施引文献: 0