环境相关浓度EHDPP长期暴露对斑马鱼的甲状腺内分泌干扰效应
Thyroid Endocrine Disruption of EHDPP at Environmentally Relevant Concentrations on Zebrafish (Danio rerio)
-
摘要: 作为一种典型的芳基有机磷阻燃剂,2-乙基己基二苯基磷酸酯(2-ethylhexyl diphenyl phosphate, EHDPP)在各种水生环境和水生生物中有着较高检出率。体外实验表明EHDPP可能通过影响甲状腺激素(thyroid hormones, TH)与转运蛋白结合进而扰乱甲状腺内分泌系统。然而目前尚未有体内实验报道其甲状腺内分泌干扰效应及可能机制。本研究中,斑马鱼胚胎(2 hours post-fertilization, 2hpf)暴露在环境相关浓度(0、1和10 μg·L-1)EHDPP中120 d,并收集F1代胚胎于清水中培养至120 hpf。研究结果表明,在F0代,EHDPP暴露显著降低成鱼存活率、体质量、肝体质常数(hepatosomatic index, HSI)和性腺体质常数(gonadosomatic index, GSI)。进一步分析发现,EHDPP显著降低雌鱼(10 μg·L-1 EHDPP暴露组)和雄鱼(1 μg·L-1 EHDPP暴露组)血清中甲状腺素(thyroxin, T4)水平,这可能在一定程度上抑制成鱼的生长。雌鱼T4水平降低伴随着促肾上腺皮质激素释放激素基因(corticotropin-releasing hormone, crh)显著性下调和尿苷二磷酸葡萄糖醛酸转移酶基因(uridine diphosphate glucuronosyl transferase, ugt1ab)显著性上调。此外,甲状腺激素转运蛋白基因(transthyretin, ttr)在1 μg·L-1和10 μg·L-1暴露组显著下调,表明EHDPP可能通过干扰甲状腺激素与甲状腺激素转运蛋白(transthyretin, TTR)结合从而影响甲状腺激素平衡。雄鱼中,甲状腺激素受体基因(thyroid receptor α, trα和thyroid receptor β, trβ)显著下调表明较少的甲状腺激素发挥作用从而抑制雄鱼生长。在F1代仔鱼中,亲代暴露于1 μg·L-1和10 μg·L-1 EHDPP分别导致三碘甲状腺原氨酸(triiodothyronin, T3)和T4水平显著降低,表明母体传递的甲状腺内分泌干扰效应。F1代仔鱼TH合成相关基因(thyroglobulin, tg)和早期甲状腺发育相关基因(paired box 8, pax8)显著下调,可能是TH水平降低的部分原因。脱碘酶基因(deiodinase 1, dio1和deiodinase 2, dio2)表达显著上调,可能是T4水平降低的代偿反应。本研究首次在体内实验中证明环境相关浓度EHDPP具有潜在甲状腺内分泌干扰效应,并且这种效应可能传递给F1代仔鱼。
-
关键词:
- 2-乙基己基二苯基磷酸酯 /
- 斑马鱼 /
- 甲状腺内分泌干扰 /
- 传递毒性
Abstract: As an aryl organophosphorus flame retardant, 2-ethylhexyl diphenyl phosphate (EHDPP) has been widely detected in various aquatic environments and organisms. Previous in vitro studies have demonstrated that EHDPP can disturb the thyroid endocrine system by affecting the binding of thyroid hormone (TH) to transport proteins. However, no in vivo studies have reported the thyroid endocrine disruption effects of EHDPP and the possible underlying mechanisms remain unknown. In this study, zebrafish (Danio rerio) embryos (2 hpf) were exposed to environmentally relevant concentrations (0, 1 and 10 μg·L-1) of EHDPP for 120 d, and the F1 generation embryos were then collected and cultured in clear water to 120 hpf. In the F0 generation, exposure to EHDPP significantly inhibited the survival rate, body weight, hepatosomatic index (HSI) and gonadosomatic index (GSI) in adult zebrafish. Further analysis revealed that 10 μg·L-1 and 1 μg·L-1 EHDPP decreased serum thyroxin (T4) levels of female and male zebrafish, respectively, which possibly contributed to the growth inhibition in adult zebrafish. The decrease of T4 levels in females was accompanied by significant down-regulation of the corticotropin-releasing hormone (crh) gene and up-regulation of the uridine diphosphate glucuronosyl transferase (ugt1ab) gene. In addition, the thyroid hormone transporter protein gene (transthyretin, ttr) was significantly downregulated in the 1 μg·L-1 and 10 μg·L-1 group, suggesting that EHDPP might affect the thyroid hormones through interfering the binding of TH to TTR. In male zebrafish, the thyroid hormone receptor genes (thyroid receptor α, trα and thyroid receptor β, trβ) were both downregulated, indicating less activities of TH, which could contribute to and resulte in the body growth inhibition. In F1 larvae, parental exposure to 1 μg·L-1 and 10 μg·L-1 EHDPP resulted in significantly decreased triiodothyronine (T3) and T4 levels in the descendants, respectively, suggesting a maternal transfer of thyroid endocrine disrupting effects by EHDPP. The expressions of TH synthesis (thyroglobulin, tg) and early thyroid development (paired box 8, pax8) related genes were significantly downregulated, which might partially be responsible for the reduced TH levels. The expressions of deiodinase genes (deiodinase 1, dio1 and deiodinase 2, dio2) were both significantly upregulated, which could possibly be a compensatory response to the decrease of T4 levels. In conclusion, we for the first time demonstrated the potential thyroid disruption effect of EHDPP at environmentally relevant concentrations in vivo, which effect could be transferred to F1 larvae. -
-
Brooke D N, Crookes M J, Quarterman P, et al. Environmental risk evaluation report:2-ethylhexyl diphenyl phosphate (CAS No. 1241-94-7)[R]. Bristol:Environment Agency, 2009:97 Wong F, de Wit C A, Newton S R. Concentrations and variability of organophosphate esters, halogenated flame retardants, and polybrominated diphenyl ethers in indoor and outdoor air in Stockholm, Sweden[J]. Environmental Pollution, 2018, 240:514-522 Cristale J, García Vázquez A, Barata C, et al. Priority and emerging flame retardants in rivers:Occurrence in water and sediment, Daphnia magna toxicity and risk assessment[J]. Environment International, 2013, 59:232-243 Christia C, Poma G, Besis A, et al. Legacy and emerging organophosphorus flame retardants in car dust from Greece:Implications for human exposure[J]. Chemosphere, 2018, 196:231-239 Yadav I C, Devi N L, Singh V K, et al. Measurement of legacy and emerging flame retardants in indoor dust from a rural village (Kopawa) in Nepal:Implication for source apportionment and health risk assessment[J]. Ecotoxicology and Environmental Safety, 2019, 168:304-314 Luo Q, Gu L Y, Wu Z P, et al. Distribution, source apportionment and ecological risks of organophosphate esters in surface sediments from the Liao River, Northeast China[J]. Chemosphere, 2020, 250:126297 Hallanger I G, Sagerup K, Evenset A, et al. Organophosphorous flame retardants in biota from Svalbard, Norway[J]. Marine Pollution Bulletin, 2015, 101(1):442-447 Zhao L M, Jian K, Su H J, et al. Organophosphate esters (OPEs) in Chinese foodstuffs:Dietary intake estimation via a market basket method, and suspect screening using high-resolution mass spectrometry[J]. Environment International, 2019, 128:343-352 Zhao F R, Wan Y, Zhao H Q, et al. Levels of blood organophosphorus flame retardants and association with changes in human sphingolipid homeostasis[J]. Environmental Science & Technology, 2016, 50(16):8896-8903 Wang X W, Liu J F, Yin Y G. Development of an ultra-high-performance liquid chromatography-tandem mass spectrometry method for high throughput determination of organophosphorus flame retardants in environmental water[J]. Journal of Chromatography A, 2011, 1218(38):6705-6711 Liu Y H, Song N H, Guo R X, et al. Occurrence and partitioning behavior of organophosphate esters in surface water and sediment of a shallow Chinese freshwater lake (Taihu Lake):Implication for eco-toxicity risk[J]. Chemosphere, 2018, 202:255-263 Xing L Q, Zhang Q, Sun X, et al. Occurrence, distribution and risk assessment of organophosphate esters in surface water and sediment from a shallow freshwater Lake, China[J]. Science of the Total Environment, 2018, 636:632-640 Sundkvist A M, Olofsson U, Haglund P. Organophosphorus flame retardants and plasticizers in marine and fresh water biota and in human milk[J]. Journal of Environmental Monitoring, 2010, 12(4):943-951 Shen J Y, Zhang Y Y, Yu N Y, et al. Organophosphate ester, 2-ethylhexyl diphenyl phosphate (EHDPP), elicits cytotoxic and transcriptomic effects in chicken embryonic hepatocytes and its biotransformation profile compared to humans[J]. Environmental Science & Technology, 2019, 53(4):2151-2160 Alzualde A, Behl M, Sipes N S, et al. Toxicity profiling of flame retardants in zebrafish embryos using a battery of assays for developmental toxicity, neurotoxicity, cardiotoxicity and hepatotoxicity toward human relevance[J]. Neurotoxicology and Teratology, 2018, 70:40-50 顾杰, 吴江, 王宏烨, 等. 有机磷酸酯对斑马鱼的早期神经毒性作用研究[J]. 生态毒理学报, 2019, 14(5):152-158 Gu J, Wu J, Wang H Y, et al. Neurotoxicity of organophosphate esters on the early life stages of zebrafish[J]. Asian Journal of Ecotoxicology, 2019, 14(5):152-158(in Chinese)
Hu W X, Gao F M, Zhang H, et al. Activation of peroxisome proliferator-activated receptor gamma and disruption of progesterone synthesis of 2-ethylhexyl diphenyl phosphate in human placental choriocarcinoma cells:Comparison with triphenyl phosphate[J]. Environmental Science & Technology, 2017, 51(7):4061-4068 Li Y, Kang Q Y, Chen R C, et al. 2-ethylhexyl diphenyl phosphate and its hydroxylated metabolites are anti-androgenic and cause adverse reproductive outcomes in male Japanese medaka (Oryzias latipes)[J]. Environmental Science & Technology, 2020, 54(14):8919-8925 Schang G, Robaire B, Hales B F. Organophosphate flame retardants act as endocrine-disrupting chemicals in MA-10 mouse tumor leydig cells[J]. Toxicological Sciences, 2016, 150(2):499-509 Cao L Y, Ren X M, Li C H, et al. Organophosphate esters bind to and inhibit estrogen-related receptorγ in cells[J]. Environmental Science & Technology Letters, 2018, 5(2):68-73 Zhao F R, Chen M, Gao F M, et al. Organophosphorus flame retardants in pregnant women and their transfer to chorionic villi[J]. Environmental Science & Technology, 2017, 51(11):6489-6497 Li R W, Yang L H, Han J, et al. Early-life exposure to tris (1,3-dichloro-2-propyl) phosphate caused multigenerational neurodevelopmental toxicity in zebrafish via altering maternal thyroid hormones transfer and epigenetic modifications[J]. Environmental Pollution, 2021, 285:117471 Kim S, Jung J, Lee I, et al. Thyroid disruption by triphenyl phosphate, an organophosphate flame retardant, in zebrafish (Danio rerio) embryos/larvae, and in GH3 and FRTL-5 cell lines[J]. Aquatic Toxicology, 2015, 160:188-196 Ma Z Y, Tang S, Su G Y, et al. Effects of tris (2-butoxyethyl) phosphate (TBOEP) on endocrine axes during development of early life stages of zebrafish (Danio rerio)[J]. Chemosphere, 2016, 144:1920-1927 Yan Z F, Feng C L, Jin X W, et al. Organophosphate esters cause thyroid dysfunction via multiple signaling pathways in zebrafish brain[J]. Environmental Science and Ecotechnology, 2022, 12:100198 Li Y, Ma H J, Chen R C, et al. Maternal transfer of 2-ethylhexyl diphenyl phosphate leads to developmental toxicity possibly by blocking the retinoic acid receptor and retinoic X receptor in Japanese medaka (Oryzias latipes)[J]. Environmental Science & Technology, 2021, 55(8):5056-5064 Yan S, Wang D Z, Teng M M, et al. Perinatal exposure to 2-ethylhexyl diphenyl phosphate (EHDPHP) affected the metabolic homeostasis of male mouse offspring:Unexpected findings help to explain dose- and diet- specific phenomena[J]. Journal of Hazardous Materials, 2020, 388:122034 Porterfield S P, Hendrich C E. The role of thyroid hormones in prenatal and neonatal neurological development-Current perspectives[J]. Endocrine Reviews, 1993, 14(1):94-106 DeGroef B, Van der Geyten S, Darras V M, et al. Role of corticotropin-releasing hormone as a thyrotropin-releasing factor in non-mammalian vertebrates[J]. General and Comparative Endocrinology, 2006, 146(1):62-68 Ortiga-Carvalho T M, Chiamolera M I, Pazos-Moura C C, et al. Hypothalamus-pituitary-thyroid axis[J]. Comprehensive Physiology, 2016, 6(3):1387-1428 Blanton M L, Specker J L. The hypothalamic-pituitary-thyroid (HPT) axis in fish and its role in fish development and reproduction[J]. Critical Reviews in Toxicology, 2007, 37(1-2):97-115 丁希胜, 马徐发, 余丽琴. 环境剂量磷酸三(1,3-二氯异丙基)酯多代暴露对斑马鱼子代仔鱼的神经发育毒性[J]. 生态毒理学报, 2020, 15(2):50-60 Ding X S, Ma X F, Yu L Q. Neurodevelopmental toxicity of zebrafish offspring after multigenerational exposure to tris(1,3-dichloro-2-propyl) phosphate at environmental concentrations[J]. Asian Journal of Ecotoxicology, 2020, 15(2):50-60(in Chinese)
Yang R Y, Wang X, Wang J W, et al. Insights into the sex-dependent reproductive toxicity of 2-ethylhexyl diphenyl phosphate on zebrafish (Danio rerio)[J]. Environment International, 2022, 158:106928 Yu L Q, Deng J, Shi X J, et al. Exposure to DE-71 alters thyroid hormone levels and gene transcription in the hypothalamic-pituitary-thyroid axis of zebrafish larvae[J]. Aquatic Toxicology, 2010, 97(3):226-233 Wang Q W, Lam J C, Man Y C, et al. Bioconcentration, metabolism and neurotoxicity of theorganophorous flame retardant 1,3-dichloro 2-propyl phosphate (TDCPP) to zebrafish[J]. Aquatic Toxicology, 2015, 158:108-115 Chen Q L, Lian X L, An J J, et al. Life cycle exposure to environmentally relevant concentrations of diphenyl phosphate (DPhP) inhibits growth and energy metabolism of zebrafish in a sex-specific manner[J]. Environmental Science & Technology, 2021, 55(19):13122-13131 Liu X S, Lu X X, Hong J B, et al. Effects of long-term exposure to TDCPP in zebrafish (Danio rerio)-Alternations of hormone balance and gene transcriptions along hypothalamus-pituitary axes[J]. Animal Models and Experimental Medicine, 2022, 5(3):239-247 Sutha J, Anila P A, Umamaheswari S, et al. Biochemical responses of a freshwater fish Cirrhinus mrigala exposed to tris(2-chloroethyl) phosphate (TCEP)[J]. Environmental Science and Pollution Research International, 2020, 27(27):34369-34387 Xu T, Wang Q W, Shi Q P, et al. Bioconcentration, metabolism and alterations of thyroid hormones of tris(1,3-dichloro-2-propyl) phosphate (TDCPP) in zebrafish[J]. Environmental Toxicology and Pharmacology, 2015, 40(2):581-586 Zeng X Y, Sun H, Huang Y Y, et al. Effects of environmentally relevant concentrations of tris (2-butoxyethyl) phosphate on growth and transcription of genes involved in the GH/IGF and HPT axes in zebrafish (Danio rerio)[J]. Chemosphere, 2018, 212:376-384 Yu L Q, Lam J C W, Guo Y Y, et al. Parental transfer of polybrominated diphenyl ethers (PBDEs) and thyroid endocrine disruption in zebrafish[J]. Environmental Science & Technology, 2011, 45(24):10652-10659 Liang X, Yu L, Gui W J, et al. Exposure to difenoconazole causes changes of thyroid hormone and gene expression levels in zebrafish larvae[J]. Environmental Toxicology and Pharmacology, 2015, 40(3):983-987 Liu X S, Cai Y, Wang Y, et al. Effects of tris(1,3-dichloro-2-propyl) phosphate (TDCPP) and triphenyl phosphate (TPP) on sex-dependent alterations of thyroid hormones in adult zebrafish[J]. Ecotoxicology and Environmental Safety, 2019, 170:25-32 Brown D D. The role of thyroid hormone in zebrafish and axolotl development[J]. Proceedings of the National Academy of Sciences of the United States of America, 1997, 94(24):13011-13016 Wang Q W, Liang K, Liu J F, et al. Exposure of zebrafish embryos/larvae to TDCPP alters concentrations of thyroid hormones and transcriptions of genes involved in the hypothalamic-pituitary-thyroid axis[J]. Aquatic Toxicology, 2013, 126:207-213 Liu S Y, Chang J H, Zhao Y, et al. Changes of thyroid hormone levels and related gene expression in zebrafish on early life stage exposure to triadimefon[J]. Environmental Toxicology and Pharmacology, 2011, 32(3):472-477 Tu W Q, Xu C, Lu B, et al. Acute exposure to synthetic pyrethroids causes bioconcentration and disruption of the hypothalamus-pituitary-thyroid axis in zebrafish embryos[J]. The Science of the Total Environment, 2016, 542(Pt A):876-885 Kim H, Ji K. Exposure to humidifier disinfectants induces developmental effects and disrupts thyroid endocrine systems in zebrafish larvae[J]. Ecotoxicology and Environmental Safety, 2019, 184:109663 Yao F, Li Y F, Ru H J, et al. Thyroid disruption and developmental toxicity caused by triphenyltin (TPT) in zebrafish embryos/larvae[J]. Toxicology and Applied Pharmacology, 2020, 394:114957 Morgado I, Santos C R, Jacinto R, et al. Regulation of transthyretin by thyroid hormones in fish[J]. General and Comparative Endocrinology, 2007, 152(2-3):189-197 Wu L Y, Li Y F, Ru H J, et al. Parental exposure to 2,2',4,4'5-pentain polybrominated diphenyl ethers (BDE-99) causes thyroid disruption and developmental toxicity in zebrafish[J]. Toxicology and Applied Pharmacology, 2019, 372:11-18 Fernie K J, Shutt J L, Mayne G, et al. Exposure to polybrominated diphenyl ethers (PBDEs):Changes in thyroid, vitamin A, glutathione homeostasis, and oxidative stress in American kestrels (Falco sparverius)[J]. Toxicological Sciences:An Official Journal of the Society of Toxicology, 2005, 88(2):375-383 Chen Q, Yu L Q, Yang L H, et al. Bioconcentration and metabolism of decabromodiphenyl ether (BDE-209) result in thyroid endocrine disruption in zebrafish larvae[J]. Aquatic Toxicology, 2012, 110-111:141-148 Orozco A, Valverde-R C. Thyroid hormone deiodination in fish[J]. Thyroid:Official Journal of the American Thyroid Association, 2005, 15(8):799-813 Li P, Li Z H, Zhong L Q. Effects of low concentrations of triphenyltin on neurobehavior and the thyroid endocrine system in zebrafish[J]. Ecotoxicology and Environmental Safety, 2019, 186:109776 Zhao X S, Wang S T, Li D M, et al. Effects of perchlorate on BDE-47-induced alteration thyroid hormone and gene expression of in the hypothalamus-pituitary-thyroid axis in zebrafish larvae[J]. Environmental Toxicology and Pharmacology, 2013, 36(3):1176-1185 Zhai W H, Huang Z G, Chen L, et al. Thyroid endocrine disruption in zebrafish larvae after exposure to mono-(2-ethylhexyl) phthalate (MEHP)[J]. PLoS One, 2014, 9(3):e92465 Liu Y R, Wu D, Xu Q L, et al. Acute exposure to tris (2-butoxyethyl) phosphate (TBOEP) affects growth and development of embryo-larval zebrafish[J]. Aquatic Toxicology, 2017, 191:17-24 Fu J J, Guo Y Y, Wang M, et al. Bioconcentration of 2,4,6-tribromophenol (TBP) and thyroid endocrine disruption in zebrafish larvae[J]. Ecotoxicology and Environmental Safety, 2020, 206:111207 Hood A, Klaassen C D. Differential effects of microsomal enzyme inducers on in vitro thyroxine (T4) and triiodothyronine (T3) glucuronidation[J]. Toxicological Sciences, 2000, 55(1):78-84 Hu F X, Zhao Y X, Yuan Y, et al. Effects of environmentally relevant concentrations of tris (2-chloroethyl) phosphate (TCEP) on early life stages of zebrafish (Danio rerio)[J]. Environmental Toxicology and Pharmacology, 2021, 83:103600 Raldúa D, Thienpont B, Babin P J. Zebrafish eleutheroembryos as an alternative system for screening chemicals disrupting the mammalian thyroid gland morphogenesis and function[J]. Reproductive Toxicology, 2012, 33(2):188-197 Porazzi P, Calebiro D, Benato F, et al. Thyroid gland development and function in the zebrafish model[J]. Molecular and Cellular Endocrinology, 2009, 312(1-2):14-23 Si W R, Zhao M J, Che H M, et al. Microcystin-LR induced transgenerational effects of thyroid disruption in zebrafish offspring by endoplasmic reticulum stress-mediated thyroglobulin accumulation and apoptosis[J]. Environmental Pollution, 2023, 322:121117 Zhao X S, Ren X, Ren B X, et al. Life-cycle exposure to BDE-47 results in thyroid endocrine disruption to adults and offsprings of zebrafish (Danio rerio)[J]. Environmental Toxicology and Pharmacology, 2016, 48:157-167 Li S Y, Wu Q, Sun Q Q, et al. Parental exposure to tebuconazole causes thyroid endocrine disruption in zebrafish and developmental toxicity in offspring[J]. Aquatic Toxicology, 2019, 211:116-123 Wang Q W, Lai N L, Wang X F, et al. Bioconcentration and transfer of the organophorous flame retardant 1,3-dichloro-2-propyl phosphate causes thyroid endocrine disruption and developmental neurotoxicity in zebrafish larvae[J]. Environmental Science & Technology, 2015, 49(8):5123-5132 Wang H K, Jing C, Peng H K, et al. Parental whole life-cycle exposure to tris (2-chloroethyl) phosphate (TCEP) disrupts embryonic development and thyroid system in zebrafish offspring[J]. Ecotoxicology and Environmental Safety, 2022, 248:114313 Zhou Y X, Fu J J, Wang M, et al. Parental and transgenerational impairments of thyroid endocrine system in zebrafish by 2,4,6-tribromophenol[J]. Journal of Environmental Sciences (China), 2023, 124:291-299 Cheng H C, Yan W, Wu Q, et al. Parental exposure to microcystin-LR induced thyroid endocrine disruption in zebrafish offspring, a transgenerational toxicity[J]. Environmental Pollution, 2017, 230:981-988 Han Z H, Li Y F, Zhang S H, et al. Prenatal transfer of decabromodiphenyl ether (BDE-209) results in disruption of the thyroid system and developmental toxicity in zebrafish offspring[J]. Aquatic Toxicology, 2017, 190:46-52 Wang X C, Ling S Y, Guan K L, et al. Bioconcentration, biotransformation, and thyroid endocrine disruption of decabromodiphenyl ethane (DBDPE), a novel brominated flame retardant, in zebrafish larvae[J]. Environmental Science & Technology, 2019, 53(14):8437-8446 Dohán O, Carrasco N. Advances in Na(+)/I(-) symporter (NIS) research in the thyroid and beyond[J]. Molecular and Cellular Endocrinology, 2003, 213(1):59-70 Noguchi Y, Harii N, Giuliani C, et al. Thyroglobulin (Tg) induces thyroid cell growth in a concentration-specific manner by a mechanism other than thyrotropin/cAMP stimulation[J]. Biochemical and Biophysical Research Communications, 2010, 391(1):890-894 Zhang S N, Guo X C, Lu S Y, et al. Exposure to PFDoA causes disruption of the hypothalamus-pituitary-thyroid axis in zebrafish larvae[J]. Environmental Pollution, 2018, 235:974-982 -
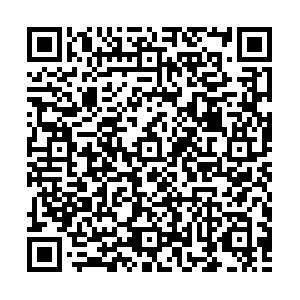
计量
- 文章访问数: 1582
- HTML全文浏览数: 1582
- PDF下载数: 132
- 施引文献: 0