2-乙基己基二苯基磷酸酯急性暴露对斑马鱼仔鱼脂质代谢和线粒体功能的影响
Effects of Acute Exposure to 2-ethylhexyl Diphenyl Phosphate on Lipid Metabolism and Mitochondrial Function in Zebrafish Larvae
-
摘要: 2-乙基己基二苯基磷酸酯(2-ethylhexyl diphenyl phosphate, EHDPP)是一种典型的有机磷阻燃剂,该化合物的大量使用导致其在环境基质中被频繁检出。大量离体研究表明EHDPP具有干扰脂质代谢的不良影响,但其是否会引起活体脂质代谢紊乱及潜在的机制尚不明确。本研究将斑马鱼胚胎(2 hours post-fertilization, 2 hpf)暴露在环境相关浓度(0、1、10、100、250和500 μg·L-1)EHDPP中5 d,评估EHDPP对仔鱼脂质代谢和线粒体功能的影响。结果表明,和对照组相比,100、250和500 μg·L-1 EHDPP暴露显著降低仔鱼孵化率、体长、心率和存活率,畸形率升高,表现出显著的生长发育毒性。结果还显示EHDPP引发脂质紊乱,总胆固醇和甘油三酯的含量在500 μg·L-1 EHDPP暴露组较对照组显著性降低。EHDPP暴露显著降低仔鱼三磷酸腺苷(adenosine triphosphate, ATP)、超氧化物歧化酶(superoxide dismutase, SOD)和谷胱甘肽(glutathione, GSH)水平,活性氧(reactive oxygen species, ROS)水平显著性升高,这提示EHDPP可能会诱发线粒体功能障碍。进一步发现EHDPP暴露导致仔鱼18rs-rrn基因表达显著性下降,而参与调控线粒体分裂(线粒体动力相关蛋白(drp1)和线粒体分裂蛋白(fis1))、融合(视神经萎缩蛋白(opa1))和自噬(PTEN诱导激酶1(pink1)和FUN14域蛋白1(fundc1))的基因表达显著性升高,表明EHDPP引发线粒体功能障碍。参与脂质合成的基因(脂肪酸合酶基因(fas)和载脂蛋白E基因(apoe))显著性降低,而调控脂质代谢的基因,如过氧化物酶体增殖物激活受体α基因(pparα)、过氧化物酶体增殖物激活受体β基因(pparβ)和酰基辅酶A氧化酶1基因(acox1)显著性升高,这些结果表明EHDPP可能引发斑马鱼线粒体功能障碍进而干扰斑马鱼幼鱼的脂肪酸氧化和脂质合成,总的来说,本研究发现EHDPP可能诱发脂质紊乱,并首次在体内实验中表明EHDPP会引发斑马鱼线粒体功能障碍,为EHDPP的毒性机制的研究提供新的思路。
-
关键词:
- 2-乙基己基二苯基磷酸酯 /
- 斑马鱼 /
- 脂质紊乱 /
- 线粒体功能障碍
Abstract: 2-ethylhexyl diphenyl phosphate (EHDPP) is an aryl organophosphorus flame retardant, which is ubiquitously found in various environmental matrices. While previous in vitro studies have emphasized the negative impact of EHDPP on lipid metabolism, there remains a dearth of in vivo research exploring its potential toxic effects and underlying mechanisms. In the present study, zebrafish (Danio rerio) embryos (<2 hpf) were exposed to environmentally relevant concentrations (0, 1, 10, 100, 250 and 500 μg·L-1) for 5 days. Results showed that 100, 250 and 500 μg·L-1 EHDPP may lead to developmental toxicity, evidenced by the increased malformation rate, reduced hatching rate, heart rate, survival rate and body length, in comparison with the control. The levels of total cholesterol and triglycerides were significantly reduced in 500 μg·L-1 EHDPP group compared to the control, suggesting that EHDPP caused disorders of lipid metabolism. EHDPP resulted in a notable reduction in the concentrations of ATP, SOD, and GSH, along with an elevation in ROS levels, suggestive of mitochondrial dysfunction triggered by EHDPP. Upon further analysis, it was revealed that the transcription of the 18rs-rrn gene was significantly reduced, while the expressions of genes involved in mitochondrial division (including mitochondrial fission protein 1 (fis1) and dynamin-related protein (drp1)), fusion (optic atrophy 1 (opa1)), and autophagy (PTEN-induced putative kinase 1 (pink1) and FUN14 domain containing 1 (fundc1)) were notably upregulated, which may partially account for the mitochondrial dysfunction. Genes involved in lipid biosynthesis including acid synthetase (fas) and apolipoprotein E (apoe) genes were significantly downregulated, whereas genes related to lipid metabolism such as peroxisome proliferators-activated receptors (pparα and pparβ) and acyl-CoA oxidase 1 (acox1) were upregulated. These results implied that EHDPP may disturb fatty acid oxidation and lipid synthesis in zebrafish larvae which might result from mitochondrial dysfunction. In conclusion, our study found that EHDPP may induce lipid metabolism disorders. For the first time, we have demonstrated mitochondrial dysfunction in vivo resulting from the effects of EHDPP, potentially offering novel insights into the toxicity elicited by this compound. -
-
van der Veen I, de Boer J. Phosphorus flame retardants: Properties, production, environmental occurrence, toxicity and analysis [J]. Chemosphere, 2012, 88(10): 1119-1153 Wong F, de Wit C A, Newton S R. Concentrations and variability of organophosphate esters, halogenated flame retardants, and polybrominated diphenyl ethers in indoor and outdoor air in Stockholm, Sweden [J]. Environmental Pollution (Barking, Essex: 1987), 2018, 240: 514-522 Violaki K, Castro-Jiménez J, Nenes A, et al. Spatial and temporal patterns of organophosphate esters flame retardants and plasticizers in airborne particles over the Mediterranean Sea [J]. Chemosphere, 2024, 348: 140746 Xie C M, Qiu N, Xie J L, et al. Organophosphate esters in seawater and sediments from the low-latitude tropical sea [J]. The Science of the Total Environment, 2024, 907: 167930 Tao F, Sjöström Y, de Wit C A, et al. Organohalogenated flame retardants and organophosphate esters from home and preschool dust in Sweden: Pollution characteristics, indoor sources and intake assessment [J]. The Science of the Total Environment, 2023, 896: 165198 Yun J, Zhang Q, Dou M S, et al. Characteristics, sources, bio-accessibility, and health risks of organophosphate esters in urban surface dust, soil, and dustfall in the arid city of Urumqi in China [J]. The Science of the Total Environment, 2024, 912: 169125 Gbadamosi M R, Moberuagba K H, Abdallah M A E, et al. Occurrence and dietary exposure to organophosphorus esters in foodstuffs of Nigerian origin [J]. Food Control, 2023, 152: 109880 Guo Y T, Chen M Q, Liao M X, et al. Organophosphorus flame retardants and their metabolites in paired human blood and urine [J]. Ecotoxicology and Environmental Safety, 2023, 268: 115696 Lee S, Cho H J, Choi W, et al. Organophosphate flame retardants (OPFRs) in water and sediment: Occurrence, distribution, and hotspots of contamination of Lake Shihwa, Korea [J]. Marine Pollution Bulletin, 2018, 130: 105-112 Wang X W, Liu J F, Yin Y G. Development of an ultra-high-performance liquid chromatography-tandem mass spectrometry method for high throughput determination of organophosphorus flame retardants in environmental water [J]. Journal of Chromatography A, 2011, 1218(38): 6705-6711 Liang C, Mo X J, Xie J F, et al. Organophosphate tri-esters and di-esters in drinking water and surface water from the Pearl River Delta, South China: Implications for human exposure [J]. Environmental Pollution, 2022, 313: 120150 Sundkvist A M, Olofsson U, Haglund P. Organophosphorus flame retardants and plasticizers in marine and fresh water biota and in human milk [J]. Journal of Environmental Monitoring, 2010, 12(4): 943-951 Aznar-Alemany Ò, Sala B, Jobst K J, et al. Temporal trends of halogenated and organophosphate contaminants in striped dolphins from the Mediterranean Sea [J]. The Science of the Total Environment, 2021, 753: 142205 Shu Y, Yuan J L, Hogstrand C, et al. Bioaccumulation and thyroid endcrione disruption of 2-ethylhexyl diphenyl phosphate at environmental concentration in zebrafish larvae [J]. Aquatic Toxicology, 2024, 267: 106815 Sun L W, Xu W B, Peng T, et al. Developmental exposure of zebrafish larvae to organophosphate flame retardants causes neurotoxicity [J]. Neurotoxicology and Teratology, 2016, 55: 16-22 Behl M, Hsieh J H, Shafer T J, et al. Use of alternative assays to identify and prioritize organophosphorus flame retardants for potential developmental and neurotoxicity [J]. Neurotoxicology and Teratology, 2015, 52(Pt B): 181-193 Yang R Y, Wang X, Wang J W, et al. Insights into the sex-dependent reproductive toxicity of 2-ethylhexyl diphenyl phosphate on zebrafish (Danio rerio) [J]. Environment International, 2022, 158: 106928 Yue J J, Sun C T, Tang J Y, et al. Downregulation of miRNA-155-5p contributes to the adipogenic activity of 2-ethylhexyl diphenyl phosphate in 3T3-L1 preadipocytes [J]. Toxicology, 2023, 487: 153452 Negi C K, Gadara D, Kohoutek J, et al. Replacement flame-retardant 2-ethylhexyldiphenyl phosphate (EHDPP) disrupts hepatic lipidome: Evidence from human 3D hepatospheroid cell culture [J]. Environmental Science & Technology, 2023, 57(5): 2006-2018 Yan S, Wang D Z, Teng M M, et al. Perinatal exposure to 2-ethylhexyl diphenyl phosphate (EHDPHP) affected the metabolic homeostasis of male mouse offspring: Unexpected findings help to explain dose- and diet- specific phenomena [J]. Journal of Hazardous Materials, 2020, 388: 122034 申屠路媚, 牟艳玲. 线粒体功能障碍机制及其相关疾病研究进展[J]. 生命科学, 2018, 30(1): 87-93 Shentu L M, Mu Y L. Research progress in mitochondrial dysfunction and its related diseases [J]. Chinese Bulletin of Life Sciences, 2018, 30(1): 87-93(in Chinese)
Ribas G S, Vargas C R. Evidence that oxidative disbalance and mitochondrial dysfunction are involved in the pathophysiology of fatty acid oxidation disorders [J]. Cellular and Molecular Neurobiology, 2022, 42(3): 521-532 Liu Y X, Liu S, Huang J, et al. Mitochondrial dysfunction in metabolic disorders induced by per- and polyfluoroalkyl substance mixtures in zebrafish larvae [J]. Environment International, 2023, 176: 107977 Yan H, Meng Y H, Li X, et al. FAM3A maintains metabolic homeostasis by interacting with F1-ATP synthase to regulate the activity and assembly of ATP synthase [J]. Metabolism: Clinical and Experimental, 2023, 139: 155372 Vaka V R, McMaster K M, Cunningham M W Jr, et al. Role of mitochondrial dysfunction and reactive oxygen species in mediating hypertension in the reduced uterine perfusion pressure rat model of preeclampsia [J]. Hypertension, 2018, 72(3): 703-711 Barbier O, Arreola-Mendoza L, Del Razo L M. Molecular mechanisms of fluoride toxicity [J]. Chemico-Biological Interactions, 2010, 188(2): 319-333 Teng J, Zhao J M, Zhu X P, et al. Oxidative stress biomarkers, physiological responses and proteomic profiling in oyster (Crassostrea gigas) exposed to microplastics with irregular-shaped PE and PET microplastic [J]. Science of the Total Environment, 2021, 786: 147425 Yu L Q, Deng J, Shi X J, et al. Exposure to DE-71 alters thyroid hormone levels and gene transcription in the hypothalamic-pituitary-thyroid axis of zebrafish larvae [J]. Aquatic Toxicology, 2010, 97(3): 226-233 Su Y Q, Luan M, Huang W, et al. Determinants of organophosphate esters exposure in pregnant women from East China [J]. Environmental Pollution, 2024, 340(Pt 1): 122767 Li Y, Kang Q Y, Chen R C, et al. 2-ethylhexyl diphenyl phosphate and its hydroxylated metabolites are anti-androgenic and cause adverse reproductive outcomes in male Japanese medaka (Oryzias latipes) [J]. Environmental Science & Technology, 2020, 54(14): 8919-8925 Ni A Y, Fang L, Xi M C, et al. Neurotoxic effects of 2-ethylhexyl diphenyl phosphate exposure on zebrafish larvae: Insight into inflammation-driven changes in early motor behavior [J]. The Science of the Total Environment, 2024, 915: 170131 Xu S Y, Yu Y X, Qin Z, et al. Effects of 2-ethylhexyl diphenyl phosphate exposure on the glucolipid metabolism and cardiac developmental toxicity in larval zebrafish based on transcriptomic analysis [J]. Comparative Biochemistry and Physiology Toxicology & Pharmacology, 2023, 267: 109578 Wang S M, Hu X G, Li X Y. Sub-chronic exposure to tris(1,3-dichloro-2-propyl) phosphate induces sex-dependent hepatotoxicity in rats [J]. Environmental Science and Pollution Research International, 2019, 26(32): 33351-33362 Henderson R J. Fatty acid metabolism in freshwater fish with particular reference to polyunsaturated fatty acids [J]. Archiv Fur Tierernahrung, 1996, 49(1): 5-22 Smith S, Witkowski A, Joshi A K. Structural and functional organization of the animal fatty acid synthase [J]. Progress in Lipid Research, 2003, 42(4): 289-317 Jin Y Z, McFie P J, Banman S L, et al. Diacylglycerol acyltransferase-2(DGAT2) and monoacylglycerol acyltransferase-2(MGAT2) interact to promote triacylglycerol synthesis [J]. The Journal of Biological Chemistry, 2014, 289(41): 28237-28248 Otis J P, Zeituni E M, Thierer J H, et al. Zebrafish as a model for apolipoprotein biology: Comprehensive expression analysis and a role for ApoA-IV in regulating food intake [J]. Disease Models & Mechanisms, 2015, 8(3): 295-309 Peng H, Chiu T Y, Liang Y J, et al. PRAP1 is a novel lipid-binding protein that promotes lipid absorption by facilitating MTTP-mediated lipid transport [J]. The Journal of Biological Chemistry, 2021, 296: 100052 Montagner A, Polizzi A, Fouché E, et al. Liver PPARα is crucial for whole-body fatty acid homeostasis and is protective against NAFLD [J]. Gut, 2016, 65(7): 1202-1214 Risérus U, Sprecher D, Johnson T, et al. Activation of peroxisome proliferator-activated receptor (PPAR)delta promotes reversal of multiple metabolic abnormalities, reduces oxidative stress, and increases fatty acid oxidation in moderately obese men [J]. Diabetes, 2008, 57(2): 332-339 Poirier Y, Antonenkov V D, Glumoff T, et al. Peroxisomal β-oxidation: A metabolic pathway with multiple functions [J]. Biochimica et Biophysica Acta-Molecular Cell Research, 2006, 1763(12): 1413-1426 马学虎, 马莉花, 苟妍, 等. 线粒体功能障碍引起的相关炎性疾病及靶向治疗[J]. 生物技术通报, 2023, 39(6): 119-125 Ma X H, Ma L H, Gou Y, et al. Related inflammatory diseases caused by mitochondrial dysfunction and targeted therapy to them [J]. Biotechnology Bulletin, 2023, 39(6): 119-125(in Chinese)
李蒙蒙, 贾岩, 王飞虾, 等. 线粒体功能障碍与慢性肝病[J]. 生理科学进展, 2018, 49(2): 81-86 Li M M, Jia Y, Wang F X, et al. Mitochondrial dysfunction and chronic liver diseases [J]. Progress in Physiological Sciences, 2018, 49(2): 81-86(in Chinese)
Schieber M, Chandel N S. ROS function in redox signaling and oxidative stress [J]. Current Biology, 2014, 24(10): R453-R462 Saquib Q, Al-Salem A M, Siddiqui M A, et al. Organophosphorus flame retardant TDCPP displays genotoxic and carcinogenic risks in human liver cells [J]. Cells, 2022, 11(2): 195 Ighodaro O, Ighodaro O, Akinloye O. First line defence antioxidants-superoxide dismutase (SOD), catalase (CAT) and glutathione peroxidase (GPX): Their fundamental role in the entire antioxidant defence grid [J]. Alexandria Journal of Medicine, 2018, 54: 287-293 顾杰, 吴江, 王宏烨, 等. 有机磷酸酯对斑马鱼的早期神经毒性作用研究[J]. 生态毒理学报, 2019, 14(5): 152-158 Gu J, Wu J, Wang H Y, et al. Neurotoxicity of organophosphate esters on the early life stages of zebrafish [J]. Asian Journal of Ecotoxicology, 2019, 14(5): 152-158(in Chinese)
Shi J, Wang X T, Chen L L, et al. HBCD, TBECH, and BTBPE exhibit cytotoxic effects in human vascular endothelial cells by regulating mitochondria function and ROS production [J]. Environmental Toxicology, 2021, 36(8): 1674-1682 Yu Y H, Xu J P, Li H, et al. α-lipoic acid improves mitochondrial biogenesis and dynamics by enhancing antioxidant and inhibiting Wnt/Ca2+ pathway to relieve fluoride-induced hepatotoxic injury [J]. Chemico-Biological Interactions, 2023, 385: 110719 Artuso L, Romano A, Verri T, et al. Mitochondrial DNA metabolism in early development of zebrafish (Danio rerio) [J]. Biochimica et Biophysica Acta, 2012, 1817(7): 1002-1011 Napoli E, Hung C, Wong S, et al. Toxicity of the flame-retardant BDE-49 on brain mitochondria and neuronal progenitor striatal cells enhanced by a PTEN-deficient background [J]. Toxicological Sciences: An Official Journal of the Society of Toxicology, 2013, 132(1): 196-210 Wu W X, Tian W L, Hu Z, et al. ULK1 translocates to mitochondria and phosphorylates FUNDC1 to regulate mitophagy [J]. EMBO Reports, 2014, 15(5): 566-575 Nguyen T N, Padman B S, Lazarou M. Deciphering the molecular signals of PINK1/parkin mitophagy [J]. Trends in Cell Biology, 2016, 26(10): 733-744 Ma X C, Lu D Z, Liu Y, et al. Multiplexed quantitative evaluation on mitochondrial toxicity of tris (2,3-dibromopropyl) phosphate in hepatocyte [J]. Ecotoxicology and Environmental Safety, 2021, 221: 112425 Le Y F, Shen H P, Yang Z, et al. Comprehensive analysis of organophosphorus flame retardant-induced mitochondrial abnormalities: Potential role in lipid accumulation [J]. Environmental Pollution (Barking, Essex: 1987), 2021, 274: 116541 -
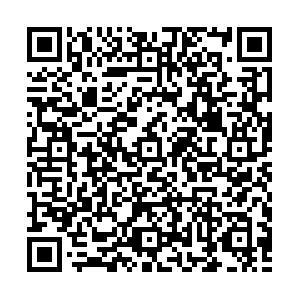
计量
- 文章访问数: 1481
- HTML全文浏览数: 1481
- PDF下载数: 157
- 施引文献: 0