纳米材料对底栖动物的毒性效应研究进展
Review on the Ecotoxicity of Manufactured Nanomaterials to the Benthic Invertebrates
-
摘要: 随着纳米技术的飞速发展,纳米材料被广泛应用于各个领域,在纳米材料生产运输、使用以及使用后的废弃过程中,纳米材料不可避免地会被释放到环境中。一旦纳米材料进入到水体,它们会经过一系列的迁移转化最终沉降在底泥中,并可能会对底栖动物产生潜在毒性效应。笔者重点总结和归纳了不同类型的常见人工纳米材料对底栖动物的毒性效应与其暴露环境和纳米材料自身物理化学特性的关系,发现在不同的毒性评价指标包括筑穴行为、生存率、发育繁殖、摄食率以及生物累积效应中,生物累积是底栖动物对纳米材料反应最为灵敏的评价指标。同时,底栖动物对纳米材料的生物累积不仅与纳米颗粒的大小和聚集程度有关,而且也与生物体内的消化微环境和和消化过程(pH、消化速率、同化效率和肠道的停留时间)相关。在归纳总结基础上,提出了完善纳米水生生物毒性效应研究的建议。Abstract: With the development of nanotechnology, nanomaterials (NMs) have been widely used in many industrial applications. During the life cycle of nanomaterial products, NMs can be released into the aquatic environment and may become toxic to benthic invertebrates. However, the complex relationships among nanomaterial physico-chemical properties, the environmental conditions, and the ecotoxicology induced by NMs in benthic invertebrates have not been studied in detail. Our review of literatures on NMs impact on benthic invertebrates found that the bioaccumulation is a more sensitive indicator to evaluate the stress as compared to burrowing behavior, mortality, reproduction and filtering ability. The bioaccumulation of NMs in the benthic invertebrates is not only correlated with the size and aggregation of NMs but also relevant to the microenvironment and digestion processes in the gut of benthic invertebrates. Our findings provide practical insights into ecotoxicology of manufactured nanomaterials in the aquatic environment.
-
Key words:
- manufactured nanomaterials /
- benthic invertebrates /
- ecotoxicity
-
-
Potocnik J. Commission Recommendation of 18 October 2011 on the definition of nanomaterial (2011/696/EU)[R]. EurepeanUnion, 2011, 275:38-40 Luis Guillermo G B, Ismael Manuel U O, Estefany Ingrid M R, et al. Difficulties in establishing regulations for engineered nanomaterials and considerations for policy makers:Avoiding an unbalance between benefits and risks[J]. Journal of Applied Toxicology, 2015, 35(10):1073-1085 Colvin V L. The potential environmental impact of engineered nano-materials[J]. Nature Biotechnology, 2003, 21(10):1166-1170 朱小山, 朱琳. 人工纳米材料生物效应研究进展[J]. 安全与环境学报, 2005, 5(4):86-90 Zhu X S, Zhu L. Review on biological effects of manufactured nano-materials[J]. Journal of Safety and Environment, 2005, 5(4):86-90(in Chinese)
Dong Y, Feng S S. In vitro and in vivo evaluation of methoxy polyethylene glycol-polylactide (MPEG-PLA) nanoparticles for small-molecule drug chemotherapy[J]. Biomaterials, 2007, 28(28):4154-4160 Nel A, Xia T, M ä dler L, et al. Toxic potential of materials at the nanolevel[J]. Science, 2006, 311(5761):622-627 陆荔, 马明, 张宇, 等. 纳米材料生物安全性研究进展[J]. 东南大学学报:自然科学版, 2004, 34(5):711-714 Lu L, Ma M, Zhang Y, et al. Development of study on the bio-safety of nanomaterials[J]. Journal of Southeast University:Natural Science Edition, 2004, 34(5):711-714(in Chinese)
Thit A, Banta G T, Selck H. Bioaccumulation, subcellular distribution and toxicity of sediment-associated copper in the ragworm Nereis diversicolor:The relative importance of aqueous copper, copper oxide nanoparticles and microparticles[J]. Environmental Pollution, 2015, 202:50-57 Zhao J, Wang Z, White J C, et al. Graphene in the aquatic environment:Adsorption, dispersion, toxicity and transformation[J]. Environmental Science & Technology, 2014, 48(17):9995-10009 Klaine S J, Alvarez P J J, Batley G E, et al. Nanomaterials in the environment:Behavior, fate, bioavailability, and effects[J]. Environmental Toxicology and Chemistry, 2008, 27(9):1825-1851 Pakarinen K, Petersen E J, Leppanen M T, et al. Adverse effects of fullerenes (nC60) spiked to sediments on Lumbriculus variegatus (Oligochaeta)[J]. Environmental Pollution, 2011, 159(12):3750-3756 Cabral S P. Effects of fullerenes on a freshwater benthic community:Toxicity and implications for ecological functions and services[D]. Rochester:Rochester Institute of Technology, 2018:1-57 Wang J, Wages M, Yu S, et al. Bioaccumulation of fullerene (C60) and corresponding catalase elevation in Lumbriculus variegatus[J]. Environmental Toxicology and Chemistry, 2014, 33(5):1135-1141 Waissi-Leinonen G C, Nybom I, Pakarinen K, et al. Fullerenes (nC60) affect the growth and development of the sediment-dwelling invertebrate Chironomus riparius larvae[J]. Environmental Pollution, 2015, 206:17-23 Waissi G C, Vaananen K, Nybom I, et al. The chronic effects of fullerene C60-associated sediments in the midge Chironomus riparius-Responses in the first and the second generation[J]. Environmental Pollution, 2017, 229:423-430 Petersen E J, Huang Q G, Weber W J. Ecological uptake and depuration of carbon nanotubes by Lumbriculus variegatus[J]. Environmental Health Perspectives, 2018, 116(4):496-500 Galloway T, Lewis C, Dolciotti I, et al. Sublethal toxicity of nano-titanium dioxide and carbon nanotubes in a sediment dwelling marine polychaete[J]. Environmental Pollution, 2010, 158(5):1748-1755 Velzeboer I, Peeters E T, Koelmans A A. Multiwalled carbon nanotubes at environmentally relevant concentrations affect the composition of benthic communities[J]. Environmental Science & Technology, 2013, 47(13):7475-7482 Marta W, Witold J, Tomasz W, et al. Toxicity of silver nanoparticles, multiwalled carbon nanotubes and dendrimers assessed with multicellular organism C. elegans[J]. Toxicology Mechanisms and Methods, 2018, 28(6):432-439 Zhang P H, Selck H, Tangaa S R, et al. Bioaccumulation and effects of sediment-associated gold- and graphene oxide nanoparticles on Tubifex tubifex[J]. Journal of Environment and Science, 2017, 51:138-145 Castro V L, Clemente Z, Jonsson C, et al. Nanoecotoxicity assessment of graphene oxide and its relationship with humic acid[J]. Environmental Toxicology and Chemistry, 2018, 37(7):1998-2012 Buffet P E, Zalouk-Vergnoux A, Châtel A, et al. A marine mesocosm study on the environmental fate of silver nanoparticles and toxicity effects on two endobenthic species:The ragworm Hediste diversicolor and the bivalve mollusk Scrobicularia plana[J]. Science of the Total Environment, 2014, 470-471:1151-1159 Cong Y, Banta G T, Selck H, et al. Toxicity and bioaccumulation of sediment-associated silver nanoparticles in the estuarine polychaete, Nereis (Hediste) diversicolor[J]. Aquatic Toxicology, 2014, 156:106-115 Wang H H, Ho K T, Scheckel K G, et al. Toxicity, bioaccumulation and biotransformation of bilver nanoparticles in marine organisms[J]. Environmental Science & Technology, 2014, 48(23):13711-13717 Carrazco-Quevedo A, Römer I, Salamanca M J, et al. Bioaccumulation and toxic effects of nanoparticulate and ionic silver in Saccostrea glomerata (rock oyster)[J]. Ecotoxicology and Environmental Safety, 2019, 179:127-134 Tangaa S R, Selck H, Winther-Nielsen M, et al. A biodynamic understanding of dietborne and waterborne Ag uptake from Ag NPs in the sediment-dwelling oligochaete, Tubifex tubifex[J]. NanoImpact, 2018, 11:33-41 Kleiven M, Rossbach L M, Gallego-Urrea J A, et al. Characterizing the behavior, uptake, and toxicity of NM300K silver nanoparticles in Caenorhabditis elegans[J]. Environmental Toxicology and Chemistry, 2018, 37(7):1799-1810 An H J, Sarkheil M, Park H S, et al. Comparative toxicity of silver nanoparticles (AgNPs) and silver nanowires (AgNWs) on saltwater microcrustacean, Artemia salina[J]. Comparative Biochemistry and Physiology, Part C, 2019, 218:62-69 Luo X, Xu S M, Yang Y N, et al. Insights into the ecotoxicity of silver nanoparticles transferred from Escherichia coli to Caenorhabditis elegans[J]. Scientific Reports, 2016, 6:36465 Lüderwald S, Schell T, Newton K, et al. Exposure pathway dependent effects of titanium dioxide and silver nanoparticles on the benthic amphipod Gammarus fossarum[J]. Aquatic Toxicity, 2019, 212:47-53 Bao S, Huang J L, Liu X, et al. Tissue distribution of Ag and oxidative stress responses in the fresh water snail Bellamya aeruginosa exposed to sediment-associated Ag nanoparticles[J]. Science of the Total Environment, 2018, 644:736-746 Echavarri-Bravo V, Paterson L, Aspray T J, et al. Shifts in the metabolic function of a benthic estuarine microbial community following a single pulse expose to silver nanoparticles[J]. Environmental Pollution, 2015, 201:91-99 Pamela J W, Nuraan K, Alaric P. The effect of biogenic and chemically manufactured silver nanoparticles on the benthic bacterial communities in river sediments[J]. Science of the Total Environment, 2018, 644:1380-1390 Jiang H S, Yin L, Ren N N, et al. The effect of chronic silver nanoparticles on aquatic system in microcosms[J]. Environmental Pollution, 2017, 223:395-402 Liu W W, Zeng Z T, Chen A, et al. Toxicity effects of silver nanoparticles on the freshwater bivalve Corbicula fluminea[J]. Journal of Environmental Chemical Engineering, 2018, 6:4236-4244 Park S Y, Chung J, Colman B P, et al. Ecotoxicity of bare and coated silver nanoparticles in the aquatic midge, Chironomus riparius[J]. Environmental Toxicology and Chemistry, 2015, 34(9):2023-2032 Nair P M, Park S Y, Choi J. Evaluation of the effect of silver nanoparticles and silver ions using stress responsive gene expression in Chironomus riparius[J]. Chemosphere, 2013, 92(5):592-599 Ahn J M, Eom H J, Yang X Y, et al. Comparative toxicity of silver nanoparticles on oxidative stress and DNA damage in the nematode, Caenorhabditis elegans[J]. Chemosphere, 2014, 108:343-352 Ale A, Liberatori G, Luisa M, et al. Exposure to a nanosilver-enabled consumer product results in similar accumulation and toxicity of silver nanoparticles in the marine mussel Mytilus galloprovincialis[J]. Aquatic Toxicology, 2019, 211:46-56 Pan J F, Buffet P E, Poirier L, et al. Size dependent bioaccumulation and ecotoxicity of gold nanoparticles in an endobenthic invertebrate:The Tellinid clam Scrobicularia plana[J]. Environmental Pollution, 2012, 168:37-43 Hudson M L, Costello D M, Daley J M, et al. Species-specific (Hyalella azteca and Lymnea stagnalis) dietary accumulation of gold nano-particles associated with periphyton[J]. Bulletin of Environmental Contamination and Toxicity, 2019, 103(2):255-260 Kay T H, Lisa P, Anthony A, et al. Effects of micronized and nano-copper azole on marine benthic communities[J]. Environmental Toxicology and Chemistry, 2018, 37(2):362-375 Thit A, Dybowska A, Kobler C, et al. Influence of copper oxide nanoparticle shape on bioaccumulation, cellular internalization and effects in the estuarine sediment-dwelling polychaete, Nereis diversicolor[J]. Marine Environmental Research, 2015, 111:89-98 Pang C F, Selck H, Banta G T, et al. Bioaccumulation, toxicokinetics, and effects of copper from sediment spiked with aqueous Cu, nano-CuO, or micro-CuO in the deposit-feeding snail, Potamopyrgus antipodarum[J]. Environmental Toxicology and Chemistry, 2013, 32(7):1561-1573 Pang C F, Selck H, Misra S K, et al. Effects of sediment-associated copper to the deposit-feeding snail, Potamopyrgus antipodarum:A comparison of Cu added in aqueous form or as nano- and micro-CuO particles[J]. Aquatic Toxicology, 2012, 106-107:114-122 Ma T W, Gong S J, Tian B. Effects of sediment-associated CuO nanoparticles on Cu bioaccumulation and oxidative stress responses in freshwater snail Bellamya aeruginosa[J]. Science of the Total Environment, 2017, 580:797-804 王萌, 马陶武, 龙奕, 等. 腐殖酸作用下沉积物中纳米氧化铜对铜锈环棱螺生态毒性的影响[J]. 生态毒理学报, 2014, 9(4):803-808 Wang M, Ma T W, Long Y, et al. Impacts of humic acid on ecotoxicity of CuO nanoparticle in Bellamya aeruginosa under the sediment scenario[J]. Asian Journal of Ecotoxicology, 2014, 9(4):803-808(in Chinese)
Lammel T, Thit A, Mouneyrac C, et al. Trophic transfer of CuO NPs and dissolved Cu from sediment to worms to fish-a proof-of-concept study[J]. Environmental Science Nano, 2019, 6:1140-1155 Marisa I, Matozzo V, Martucci A, et al. Bioaccumulation and effects of titanium dioxide nanoparticles and bulk in the clam Ruditapes philippinarum[J]. Marine Environmental Research, 2018, 136:179-189 Auguste M, Lasa A, Pallavicini A, et al. Exposure to TiO2 nanoparticles induces shifts in the microbiota composition of Mytilus galloprovincialis hemolymph[J]. Science of the Total Environment, 2019, 670:129-137 Guan X F, Tang Y, Zha S J, et al. Exogenous Ca2+ mitigates the toxic effects of TiO2 nanoparticles on phagocytosis, cell viability, and apoptosis in haemocytes of a marine bivalve mollusk, Tegillarca granosa[J]. Environmental Pollution, 2019, 252:1764-1771 Saidani W, Sellami B, Khazri A, et al. Metal accumulation, biochemical and behavioral responses on the Mediterranean clams Ruditapes decussatus exposed to two photocatalyst nanocomposites (TiO2 NPs and AuTiO2NPs)[J]. Aquatic Toxicology, 2019, 208:71-79 Nunes S M, Josende M E, Ruas C P, et al. Biochemical responses induced by co-exposition to arsenic and titanium dioxide nanoparticles in the estuarine polychaete Laeonereis acuta[J]. Toxicology, 2017, 376:51-58 Camilla D T, Teresa B, Giacomo G, et al. Titanium dioxide nanoparticles modulate the toxicological response to cadmium in the gills of Mytilus galloprovincialis[J]. Journal of Hazardous Materials, 2015, 297:92-100 Matouke M M, Mustapha M. Bioaccumulation and physiological effects of Copepods sp. (Eucyclop sp.) fed Chlorella ellipsoides exposed to titanium dioxide (TiO2) nanoparticles and lead (Pb2+)[J]. Aquatic Toxicology, 2018, 198:30-39 Wang J, Dai H, Nie Y, et al. TiO2 nanoparticles enhance bioaccumulation and toxicity of heavy metals in Caenorhabditis elegans via modification of local concentrations during the sedimentation process[J]. Ecotoxicology and Environmental Safety, 2018, 162:160-169 Huang C W, Li S W, Liao V H C. Long-term sediment exposure to ZnO nanoparticles induces oxidative stress in Caenorhabditis elegans[J]. Environmental Science Nano, 2019, 6:2602-2614 Poynton H C, Chen C, Alexander S L, et al. Enhanced toxicity of environmentally transformed ZnO nanoparticles relative to Zn ions in the epibenthic amphipod Hyalella azteca[J]. Environmental Science Nano, 2019, 6:325-340 Izabela J, Patryk O, Ewa S. The bioavailability and toxicity of ZnO and Ni nanoparticles and their bulk counterparts in different sediments[J]. Journal of Soils & Sediments, 2016, 16(6):1798-1808 Cross R K, Tyler C R, Galloway T S. The fate of cerium oxide nanoparticles in sediments and their routes of uptake in a freshwater worm[J]. Nanotoxicology, 2019, 13(7):894-908 Niemuth N J, Curtis B J, Hang M N, et al. Next-generation complex metal oxide nanomaterials negatively impact growth and development in the benthic invertebrate Chironomus riparius upon settling[J]. Environmental Science & Technology, 2019, 53(7):3860-3870 Li X, Liu W, Sun L, et al. Effects of physicochemical properties of nanomaterials on their toxicity[J]. Journal of Biomedical Materials Research. Part A, 2015, 103(7):2499-2507 Hull M S, Chaurand P, Rose J, et al. Filter-feeding bivalves store and biodeposit colloidally stable gold nanoparticles[J]. Environmental Science & Technology, 2011, 45(15):6592-6599 Ramskov T, Selck H, Banta G, et al. Bioaccumulation and effects of different-shaped copper oxide nanoparticles in the deposit-feeding snail Potamopyrgus antipodarum[J]. Environmental Toxicology and Chemistry, 2014, 33(9):1976-1987 Buffet P E, Claude A T, Agnieszka D, et al. Fate of isotopically labeled zinc oxide nanoparticles in sediment and effects on two endobenthic species, the clam Scrobicularia plana and the ragworm Hediste diversicolor[J]. Ecotoxicology and Environmental Safety, 2012, 84:191-198 Fabrega J, Tantra R, Asmer A, et al. Sequestration of zinc from zinc oxide nanoparticles and life cycle effects in the sediment dweller amphipod Corophium volutator[J]. Environmental Science & Technology, 2012, 46(2):1128-1135 Fairbairn E A, Keller A A, Mädler L, et al. Metal oxide nanomaterials in seawater:Linking physicochemical characteristics with biological response in sea urchin development[J]. Journal of Hazardous Materials, 2011, 192(3):1565-1571 Miller R J, Lenihan H S, Muller E B, et al. Impacts of metal oxide nanoparticles on marine phytoplankton[J]. Environmental Science & Technology, 2010, 44(19):7329-7334 Khan F R, Misra S K, Bury N R, et al. Inhibition of potential uptake pathways for silver nanoparticles in the estuarine snail Peringia ulvae[J]. Nanotoxicology Information Healthcare, 2015, 9(4):493-501 Dai L, Syberg K, Banta G T, et al. Effects, uptake, and depuration kinetics of silver oxide and copper oxide nanoparticles in a marine deposit feeder, Macoma balthica[J]. ACS Sustainable Chemistry & Engineering, 2013, 1(7):760-767 Buffet P E, Richard M, Caupos F, et al. A mesocosm study of fate and effects of CuO nanoparticles on endobenthic species (Scrobicularia plana, Hediste diversicolor)[J]. Environmental Science & Technology, 2013, 47:1620-1628 Buffet P E, Pan J F, Poirier L, et al. Biochemical and behavioural responses of the endobenthic bivalve Scrobicularia plana to silver nanoparticles in seawater and microalgal food[J]. Ecotoxicology and Environmental Safety, 2013, 89:117-124 Ramskov T, Forbes V E, Gilliland D, et al. Accumulation and effects of sediment-associated silver nanoparticles to sediment-dwelling invertebrates[J]. Aquatic Toxicology, 2015, 166:96-105 Bundschuh M, Seitz F, Rosenfeldt R R, et al. Effects of nanoparticles in fresh waters:Risks, mechanisms and interactions[J]. Freshwater Biology, 2016, 61:2185-2196 Li S, Wallis L K, Ma H, et al. Phototoxicity of TiO2 nanoparticles to a freshwater benthic amphipod:Are benthic systems at risk[J]. The Science of the Total Environment, 2014, 466-467:800-808 Ma H, Kabengi N J, Bertsch P M, et al. Comparative phototoxicity of nanoparticulate and bulk ZnO to a free-living nematode Caenorhabditis elegans:The importance of illumination mode and primary particle size[J]. Environmental Pollution, 2011, 159(6):1473-1480 Lowery G V, Gregory K B, Apte S C, et al. Transformations of nanomaterials in the environment[J]. Environmental Science & Technology, 2012, 46:6893-6899 Hyung H, Kim J H. Natural organic matter adsorption to multi-walled carbon nanotubes:Effect of NOM characteristics and water quality parameters[J]. Environmental Science & Technology, 2008, 42(12):4416-4421 Delay M, Dolt T, Woellhaf A, et al. Interactions and stability of silver nanoparticles in the aqueous phase:Influence of natural organic matter (NOM) and ionic strength[J]. Journal of Chromatography A, 2011, 1218(27):4206-4212 Lynch I, Dawson K A. Protein-nanoparticle interactions[J]. Nano Today, 2008, 3:40-47 Wormington A M, Coral J, Alloy M M, et al. Effect of natural organic matter on the photo-induced toxicity of titanium dioxide nanoparticles[J]. Environmental Toxicology and Chemistry, 2017, 36(6):1661-1666 Rajala J E, Vehniainen E R, Vaisanen A, et al. Toxicity of silver nanoparticles to Lumbriculus variegatus is a function of dissolved silver and promoted by low sediment pH[J]. Environmental Toxicology and Chemistry, 2018, 37(7):1889-1897 -
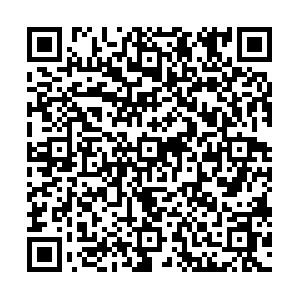
计量
- 文章访问数: 2348
- HTML全文浏览数: 2348
- PDF下载数: 95
- 施引文献: 0