纳米颗粒对淡水藻类生长的影响:毒性机制与复合毒性
Effects of Nanoparticles on Growth of Freshwater Algae: Toxicity Mechanism and Combined Toxicity
-
摘要: 近年来,纳米颗粒在生活、工业生产中的应用日益广泛,而这些纳米颗粒的应用引起的一系列环境问题越来越被密切关注。纳米材料在使用过后不可避免地会释放到水环境中,不仅会影响水生生物的生长代谢,也会污染水体,影响水源水质。而藻类作为水生食物链的初级生产者,对于纳米颗粒在水环境中的积累和迁移起着至关重要的作用。本文首先总结了不同种类的纳米颗粒对水环境中不同藻类生长代谢的影响和相关的毒性机制,包括破坏细胞完整性、氧化应激胁迫、破坏光合系统、基因水平异常和有毒物质的释放等。其次,系统总结了纳米颗粒表面特性(如粒径、晶型、表面电荷、亲疏水性、光敏性、表面涂覆、老化和纳米颗粒的均相与非均相等)、水环境影响因素(如自然有机物质、环境胶体、离子强度、pH、硬度、光照和温度等)和藻类胞外聚合物对纳米材料毒性的影响。最后,还综述了水环境中关键污染物和纳米颗粒对藻类的复合毒性。对于纳米颗粒对水环境中藻类生长的毒性作用、影响机制以及复合毒性的系统总结,有利于全面了解纳米颗粒的环境行为和生物毒性。Abstract: Recently, as nanoparticles have been increasingly applied in producing and living activities, a series of environmental problems caused by these nanoparticles have attracted more and more attentions. Nanoparticles released into aquatic environments can not only affect the growth and metabolism of aquatic organisms, but also pollute the water. As primary producer of aquatic food chain, algae play necessary roles in the accumulation and migration of nanoparticles in aquatic environment. In this paper, the effects of different kinds of nanoparticles on the growth of different algae in water environment and relevant toxicity mechanisms (such as destruction of cell integrity, oxidative stress, destruction of photosynthetic system, abnormal gene level and release of toxic substances, etc.) were summarized at first. Secondly, the influences of surface characteristics of nanoparticles (such as particle size, crystal form, surface charge, hydrophobicity, photosensitivity, surface coating, aging and homogeneous and heterogeneous type, etc.), aquatic environmental factors (such as natural organic matters, environmental colloid, ionic strength, pH, hardness, illumination, temperature, etc.), and algae extracellular polymers on the toxicity of nanoparticles were systematically discussed. Finally, the combined toxicity of typical environmental pollutants and nanoparticles to algae were reviewed. Overall, this paper thoroughly summarized the toxicity, influence mechanism and combined toxicity of nanoparticles to algae growth, which is in favor of understanding their transport and biotoxicity in aquatic environments.
-
Key words:
- nanoparticles /
- algae /
- toxicity mechanisms /
- influencing factors /
- combined toxicity
-
-
Wang F, Guan W, Xu L, et al. Effects of nanoparticles on algae:Adsorption, distribution, ecotoxicity and fate[J]. Applied Sciences, 2019, 9(8):1534 Georgantzopoulou A, Balachandran Y L, Rosenkranz P, et al. Ag nanoparticles:Size- and surface-dependent effects on model aquatic organisms and uptake evaluation with NanoSIMS[J]. Nanotoxicology, 2012, 7(7):1168-1178 倪方方, 王博林, 宋腾蛟, 等. 纳米银颗粒的毒性效应及作用机制研究进展[J]. 中国药理学通报, 2016, 32(5):593-598 Ni F F, Wang B L, Song T J, et al. Toxic effect and mechanism of silver nanoparticles[J]. Chinese Pharmacological Bulletin, 2016, 32(5):593-598(in Chinese)
Zhang S J, Jiang Y L, Chen C S, et al. Aggregation, dissolution, and stability of quantum dots in marine environments:Importance of extracellular polymeric substances[J]. Environmental Science & Technology, 2012, 46(16):8764-8772 Wu Q, Yao L, Zhao X, et al. Cellular uptake of few-layered black phosphorus and the toxicity to an aquatic unicellular organism[J]. Environmental Science & Technology, 2020, 54(3):1583-1592 Suresh A K, Pelletier D A, Doktycz M J. Relating nanomaterial properties and microbial toxicity[J]. Nanoscale, 2013, 5(2):463-474 Mao Y F, Li H, Huangfu X L, et al. Nanoplastics display strong stability in aqueous environments:Insights from aggregation behaviour and theoretical calculations[J]. Environmental Pollution, 2020, 258:113760 Deshmukh S P, Patil S M, Mullani S B, et al. Silver nanoparticles as an effective disinfectant:A review[J]. Materials Science & Engineering C, Materials for Biological Applications, 2019, 97:954-965 Trojanowski R, Fthenakis V. Nanoparticle emissions from residential wood combustion:A critical literature review, characterization, and recommendations[J]. Renewable and Sustainable Energy Reviews, 2019, 103:515-528 Chen C Y, Huang W L. Aggregation kinetics of diesel soot nanoparticles in wet environments[J]. Environmental Science & Technology, 2017, 51(4):2077-2086 Bondarenko O M, Heinlaan M, Sihtmäe M, et al. Multilaboratory evaluation of 15 bioassays for (eco)toxicity screening and hazard ranking of engineered nanomaterials:FP7 project NANOVALID[J]. Nanotoxicology, 2016, 10(9):1229-1242 Li K, Qian J, Wang P F, et al. Toxicity of three crystalline TiO2 nanoparticles in activated sludge:Bacterial cell death modes differentially weaken sludge dewaterability[J]. Environmental Science & Technology, 2019, 53(8):4542-4555 Zhang F, Wang Z, Song L, et al. Aquatic toxicity of iron-oxide-doped microplastics to Chlorella pyrenoidosa and Daphnia magna[J]. Environmental Pollution, 2020, 257:113451 Lekamge S, Miranda A F, Trestrail C, et al. The toxicity of nonaged and aged coated silver nanoparticles to freshwater alga Raphidocelis subcapitata[J]. Environmental Toxicology and Chemistry, 2019, 38(11):2371-2382 Bellingeri A, Bergami E, Grassi G, et al. Combined effects of nanoplastics and copper on the freshwater alga Raphidocelis subcapitata[J]. Aquatic Toxicology, 2019, 210:179-187 Simeone F C, Costa A L. Assessment of cytotoxicity of metal oxide nanoparticles on the basis of fundamental physical-chemical parameters:A robust approach to grouping[J]. Environmental Science:Nano, 2019, 6(10):3102-3112 Fan G D, You Y F, Wang B, et al. Inactivation of harmful cyanobacteria by Ag/AgCl@ZIF-8 coating under visible light:Efficiency and its mechanisms[J]. Applied Catalysis B:Environmental, 2019, 256:117866 Fan G D, Bao M C, Zheng X M, et al. Growth inhibition of harmful cyanobacteria by nanocrystalline Cu-MOF-74:Efficiency and its mechanisms[J]. Journal of Hazardous Materials, 2019, 367:529-538 Avellan A, Simonin M, Anderson S M, et al. Differential reactivity of copper- and gold-based nanomaterials controls their seasonal biogeochemical cycling and fate in a freshwater wetland mesocosm[J]. Environmental Science & Technology, 2020, 54(3):1533-1544 Gilroy K D, Neretina S, Sanders R W. Behavior of gold nanoparticles in an experimental algal-zooplankton food chain[J]. Journal of Nanoparticle Research, 2014, 16(5):1-8 Zhang H, Chen Z, Huang Q. Study of the toxicity of ZnO nanoparticles to Chlorella sorokiniana under the influence of phosphate:Spectroscopic quantification, photosynthetic efficiency and gene expression analysis[J]. Environmental Science:Nano, 2020, 7(5):1431-1443 Dalai S, Pakrashi S, Joyce Nirmala M, et al. Cytotoxicity of TiO2 nanoparticles and their detoxification in a freshwater system[J]. Aquatic Toxicology, 2013, 138-139:1-11 Zhao J, Dai Y H, Wang Z Y, et al. Toxicity of GO to freshwater algae in the presence of Al2O3 particles with different morphologies:Importance of heteroaggregation[J]. Environmental Science & Technology, 2018, 52(22):13448-13456 Zhang L Q, Lei C, Yang K, et al. Cellular response of Chlorella pyrenoidosa to oxidized multi-walled carbon nanotubes[J]. Environmental Science:Nano, 2018, 5(10):2415-2425 Xu Y H, He Q, Liu C H, et al. Are micro- or nanoplastics leached from drinking water distribution systems?[J]. Environmental Science & Technology, 2019, 53(16):9339-9340 Mao Y F, Ai H N, Chen Y, et al. Phytoplankton response to polystyrene microplastics:Perspective from an entire growth period[J]. Chemosphere, 2018, 208:59-68 Zhang Y, Yang R X, Si X H, et al. The adverse effect of biochar to aquatic algae-The role of free radicals[J]. Environmental Pollution, 2019, 248:429-437 Behra R, Wagner B, Sgier L, et al. Colloidal stability and toxicity of gold nanoparticles and gold chloride on Chlamydomonas reinhardtii[J]. Aquatic Geochemistry, 2015, 21(2-4):331-342 Zhang J L, Zhou Z P, Pei Y, et al. Metabolic profiling of silver nanoparticle toxicity in Microcystis aeruginosa[J]. Environmental Science:Nano, 2018, 5(11):2519-2530 Zhang J L, Xiang Q Q, Shen L, et al. Surface charge-dependent bioaccumulation dynamics of silver nanoparticles in freshwater algae[J]. Chemosphere, 2020, 247:125936 Bhuvaneshwari M, Iswarya V, Archanaa S, et al. Cytotoxicity of ZnO NPs towards fresh water algae Scenedesmus obliquus at low exposure concentrations in UV-C, visible and dark conditions[J]. Aquatic Toxicology, 2015, 162:29-38 Chen P Y, Powell B A, Mortimer M, et al. Adaptive interactions between zinc oxide nanoparticles and Chlorella sp.[J]. Environmental Science & Technology, 2012, 46(21):12178-12185 Zhou H, Wang X J, Zhou Y, et al. Evaluation of the toxicity of ZnO nanoparticles to Chlorella vulgaris by use of the chiral perturbation approach[J]. Analytical and Bioanalytical Chemistry, 2014, 406(15):3689-3695 Xia B, Chen B J, Sun X M, et al. Interaction of TiO2 nanoparticles with the marine microalga Nitzschia closterium:Growth inhibition, oxidative stress and internalization[J]. Science of the Total Environment, 2015, 508:525-533 Middepogu A, Hou J, Gao X, et al. Effect and mechanism of TiO2 nanoparticles on the photosynthesis of Chlorella pyrenoidosa[J]. Ecotoxicology and Environmental Safety, 2018, 161:497-506 Melegari S P, Perreault F, Costa R H R, et al. Evaluation of toxicity and oxidative stress induced by copper oxide nanoparticles in the green alga Chlamydomonas reinhardtii[J]. Aquatic Toxicology, 2013, 142-143:431-440 Fathi P, Sadeghi G, Hosseini M J, et al. Effects of copper oxide nanoparticles on the Chlorella algae in the presence of humic acid[J]. SN Applied Sciences, 2020, 2(2):1-11 Martín-de-Lucía I, Campos-Mañas M C, Agüera A, et al. Combined toxicity of graphene oxide and wastewater to the green alga Chlamydomonas reinhardtii[J]. Environmental Science:Nano, 2018, 5(7):1729-1744 Sendra M, Staffieri E, Yeste M P, et al. Are the primary characteristics of polystyrene nanoplastics responsible for toxicity and ad/absorption in the marine diatom Phaeodactylum tricornutum?[J]. Environmental Pollution, 2019, 249:610-619 Morelli E, Cioni P, Posarelli M, et al. Chemical stability of CdSe quantum dots in seawater and their effects on a marine microalga[J]. Aquatic Toxicology, 2012, 122-123:153-162 Akhavan O, Ghaderi E. Enhancement of antibacterial properties of Ag nanorods by electric field[J]. Science and Technology of Advanced Materials, 2009, 10(1):015003 Bundschuh M, Seitz F, Rosenfeldt R R, et al. Effects of nanoparticles in fresh waters:Risks, mechanisms and interactions[J]. Freshwater Biology, 2016, 61(12):2185-2196 王震宇, 赵建, 李娜, 等. 人工纳米颗粒对水生生物的毒性效应及其机制研究进展[J]. 环境科学, 2010, 31(6):1409-1418 Wang Z Y, Zhao J, Li N, et al. Review of ecotoxicity and mechanism of engineered nanoparticles to aquatic organisms[J]. Environmental Science, 2010, 31(6):1409-1418(in Chinese)
陈春英, 徐莺莺, 王鹏. 纳米材料的毒理学效应及其关键影响因素[J]. 中国药理学与毒理学杂志, 2013, 2(suppl.):6-13 Pakrashi S, Dalai S, Prathna T C, et al. Cytotoxicity of aluminium oxide nanoparticles towards fresh water algal isolate at low exposure concentrations[J]. Aquatic Toxicology, 2013, 132-133:34-45 Lei C, Zhang L Q, Yang K, et al. Toxicity of iron-based nanoparticles to green algae:Effects of particle size, crystal phase, oxidation state and environmental aging[J]. Environmental Pollution, 2016, 218:505-512 Wang Z Y, Li J, Zhao J, et al. Toxicity and internalization of CuO nanoparticles to prokaryotic alga Microcystis aeruginosa as affected by dissolved organic matter[J]. Environmental Science & Technology, 2011, 45(14):6032-6040 Pereira M M, Mouton L, Yéprémian C, et al. Ecotoxicological effects of carbon nanotubes and cellulose nanofibers in Chlorella vulgaris[J]. Journal of Nanobiotechnology, 2014, 12(1):1-13 Hu X G, Lu K C, Mu L, et al. Interactions between graphene oxide and plant cells:Regulation of cell morphology, uptake, organelle damage, oxidative effects and metabolic disorders[J]. Carbon, 2014, 80:665-676 Larguinho M, Correia D, Diniz M S, et al. Evidence of one-way flow bioaccumulation of gold nanoparticles across two trophic levels[J]. Journal of Nanoparticle Research, 2014, 16(8):1-11 Anand A, Unnikrishnan B, Wei S C, et al. Graphene oxide and carbon dots as broad-spectrum antimicrobial agents-A minireview[J]. Nanoscale Horizons, 2019, 4(1):117-137 Zhao J, Ning F Y, Cao X S, et al. Photo-transformation of graphene oxide in the presence of co-existing metal ions regulated its toxicity to freshwater algae[J]. Water Research, 2020, 176:115735 Chen X H, Zhang C, Tan L J, et al. Toxicity of Co nanoparticles on three species of marine microalgae[J]. Environmental Pollution, 2018, 236:454-461 Li X M, Schirmer K, Bernard L, et al. Silver nanoparticle toxicity and association with the alga Euglena gracilis[J]. Environmental Science:Nano, 2015, 2(6):594-602 Nespolo M. Free radicals in biology and medicine[J]. Acta Crystallographica Section D Structural Biology, 2017, 73(4):384-385 Sadiq I M, Pakrashi S, Chandrasekaran N, et al. Studies on toxicity of aluminum oxide (Al2O3) nanoparticles to microalgae species:Scenedesmus sp. and Chlorella sp.[J]. Journal of Nanoparticle Research, 2011, 13(8):3287-3299 Wei C X, Zhang Y B, Guo J, et al. Effects of silica nanoparticles on growth and photosynthetic pigment contents of Scenedesmus obliquus[J]. Journal of Environmental Sciences, 2010, 22(1):155-160 Barreto D M, Lombardi A T. Environmentally relevant concentrations of TiO2 nanoparticles affected cell viability and photosynthetic yield in the chlorophyceae Scenedesmus bijugus[J]. Water, Air, & Soil Pollution, 2016, 227(12):1-11 Huang Y W, Cambre M, Lee H J. The toxicity of nanoparticles depends on multiple molecular and physicochemical mechanisms[J]. International Journal of Molecular Sciences, 2017, 18(12):2702 Ouyang K, Walker S L, Yu X Y, et al. Metabolism, survival, and gene expression of Pseudomonas putida to hematite nanoparticles mediated by surface-bound humic acid[J]. Environmental Science:Nano, 2018, 5(3):682-695 Chen Y, Xu M, Zhang J, et al. Genome-wide DNA methylation variations upon exposure to engineered nanomaterials and their implications in nanosafety assessment[J]. Advanced Materials, 2017, 29(6):1604580 Zhang J, Guo W L, Li Q Q, et al. The effects and the potential mechanism of environmental transformation of metal nanoparticles on their toxicity in organisms[J]. Environmental Science:Nano, 2018, 5(11):2482-2499 Maurer-Jones M A, Mousavi M P S, Chen L D, et al. Characterization of silver ion dissolution from silver nanoparticles using fluorous-phase ion-selective electrodes and assessment of resultant toxicity to Shewanella oneidensis[J]. Chemical Science, 2013, 4(6):2564 Shao Z S, Wang W X. Biodynamics of silver nanoparticles in an estuarine oyster revealed by 110mAgNP tracing[J]. Environmental Science & Technology, 2020, 54(2):965-974 He X X, Xie C J, Ma Y H, et al. Size-dependent toxicity of ThO2 nanoparticles to green algae Chlorella pyrenoidosa[J]. Aquatic Toxicology, 2019, 209:113-120 Wan J K, Chu W L, Kok Y Y, et al. Assessing the toxicity of copper oxide nanoparticles and copper sulfate in a tropical Chlorella[J]. Journal of Applied Phycology, 2018, 30(6):3153-3165 Feng L J, Sun X D, Zhu F P, et al. Nanoplastics promote microcystin synthesis and release from cyanobacterial Microcystis aeruginosa[J]. Environmental Science & Technology, 2020, 54(6):3386-3394 Tinwala H, Wairkar S. Production, surface modification and biomedical applications of nanodiamonds:A sparkling tool for theranostics[J]. Materials Science and Engineering:C, 2019, 97:913-931 Wang J, Wang W X. Significance of physicochemical and uptake kinetics in controlling the toxicity of metallic nanomaterials to aquatic organisms[J]. Journal of Zhejiang University:Science A, 2014, 15(8):573-592 Navarro E, Baun A, Behra R, et al. Environmental behavior and ecotoxicity of engineered nanoparticles to algae, plants, and fungi[J]. Ecotoxicology, 2008, 17(5):372-386 Rudramurthy G R, Swamy M K. Potential applications of engineered nanoparticles in medicine and biology:An update[J]. JBIC Journal of Biological Inorganic Chemistry, 2018, 23(8):1185-1204 Qu C C, Qian S F, Chen L, et al. Size-dependent bacterial toxicity of hematite particles[J]. Environmental Science & Technology, 2019, 53(14):8147-8156 Wang B, Yin J J, Zhou X Y, et al. Physicochemical origin for free radical generation of iron oxide nanoparticles in biomicroenvironment:Catalytic activities mediated by surface chemical states[J]. The Journal of Physical Chemistry C, 2012, 117(1):383-392 Nolte T M, Hartmann N B, Kleijn J M, et al. The toxicity of plastic nanoparticles to green algae as influenced by surface modification, medium hardness and cellular adsorption[J]. Aquatic Toxicology, 2017, 183:11-20 Miller R J, Bennett S, Keller A A, et al. TiO2 nanoparticles are phototoxic to marine phytoplankton[J]. PLoS One, 2012, 7(1):e30321 Kalman J, Paul K B, Khan F R, et al. Characterisation of bioaccumulation dynamics of three differently coated silver nanoparticles and aqueous silver in a simple freshwater food chain[J]. Environmental Chemistry, 2015, 12(6):662-672 Salas P, Odzak N, Echegoyen Y, et al. The role of size and protein shells in the toxicity to algal photosynthesis induced by ionic silver delivered from silver nanoparticles[J]. Science of the Total Environment, 2019, 692:233-239 Perreault F, Oukarroum A, Melegari S P, et al. Polymer coating of copper oxide nanoparticles increases nanoparticles uptake and toxicity in the green alga Chlamydomonas reinhardtii[J]. Chemosphere, 2012, 87(11):1388-1394 Lin D H, Ji J, Long Z F, et al. The influence of dissolved and surface-bound humic acid on the toxicity of TiO2 nanoparticles to Chlorella sp.[J]. Water Research, 2012, 46(14):4477-4487 Foroozandeh P, Aziz A A, Mahmoudi M. Effect of cell age on uptake and toxicity of nanoparticles:The overlooked factor at the nanobio interface[J]. ACS Applied Materials & Interfaces, 2019, 11(43):39672-39687 Miao A J, Zhang X Y, Luo Z P, et al. Zinc oxide-engineered nanoparticles:Dissolution and toxicity to marine phytoplankton[J]. Environmental Toxicology and Chemistry, 2010, 29(12):2814-2822 Tejamaya M, Römer I, Merrifield R C, et al. Stability of citrate, PVP, and PEG coated silver nanoparticles in ecotoxicology media[J]. Environmental Science & Technology, 2012, 46(13):7011-7017 Liu J, Zhang T, Tian L, et al. Aging significantly affects mobility and contaminant-mobilizing ability of nanoplastics in saturated loamy sand[J]. Environmental Science & Technology, 2019, 53(10):5805-5815 Wang Y, Zhang W, Shang J, et al. Chemical aging changed aggregation kinetics and transport of biochar colloids[J]. Environmental Science & Technology, 2019, 53(14):8136-8146 范功端, 陈薇, 郑小梅, 等. 纳米材料对藻细胞毒性效应及致毒机理[J]. 生态毒理学报, 2018, 13(2):23-33 Fan G D, Chen W, Zheng X M, et al. The cytotoxic effects of nanomaterials on algae and its mechanisms[J]. Asian Journal of Ecotoxicology, 2018, 13(2):23-33(in Chinese)
Huangfu X L, Ma C X, Huang R X, et al. Deposition kinetics of colloidal manganese dioxide onto representative surfaces in aquatic environments:The role of humic acid and biomacromolecules[J]. Environmental Science & Technology, 2019, 53(1):146-156 Thio B J R, Zhou D X, Keller A A. Influence of natural organic matter on the aggregation and deposition of titanium dioxide nanoparticles[J]. Journal of Hazardous Materials, 2011, 189(1-2):556-563 Liu X Y, Wazne M, Han Y, et al. Effects of natural organic matter on aggregation kinetics of boron nanoparticles in monovalent and divalent electrolytes[J]. Journal of Colloid and Interface Science, 2010, 348(1):101-107 Mudunkotuwa I A, Pettibone J M, Grassian V H. Environmental implications of nanoparticle aging in the processing and fate of copper-based nanomaterials[J]. Environmental Science & Technology, 2012, 46(13):7001-7010 Adegboyega N F, Sharma V K, Siskova K, et al. Interactions of aqueous Ag+ with fulvic acids:Mechanisms of silver nanoparticle formation and investigation of stability[J]. Environmental Science & Technology, 2013, 47(2):757-764 Levard C, Hotze E M, Lowry G V, et al. Environmental transformations of silver nanoparticles:Impact on stability and toxicity[J]. Environmental Science & Technology, 2012, 46(13):6900-6914 Yu S J, Liu J F, Yin Y G, et al. Interactions between engineered nanoparticles and dissolved organic matter:A review on mechanisms and environmental effects[J]. Journal of Environmental Sciences, 2018, 63:198-217 Huang B, Wei Z B, Yang L Y, et al. Combined toxicity of silver nanoparticles with hematite or plastic nanoparticles toward two freshwater algae[J]. Environmental Science & Technology, 2019, 53(7):3871-3879 Ouyang S H, Zhou Q X, Zeng H, et al. Natural nanocolloids mediate the phytotoxicity of graphene oxide[J]. Environmental Science & Technology, 2020, 54(8):4865-4875 Saleh N, Kim H J, Phenrat T, et al. Ionic strength and composition affect the mobility of surface-modified Fe0 nanoparticles in water-saturated sand columns[J]. Environmental Science & Technology, 2008, 42:3349-3355 Bian S W, Mudunkotuwa I A, Rupasinghe T, et al. Aggregation and dissolution of 4 nm ZnO nanoparticles in aqueous environments:Influence of pH, ionic strength, size, and adsorption of humic acid[J]. Langmuir, 2011, 27(10):6059-6068 Cupi D, Hartmann N B, Baun A. Influence of pH and media composition on suspension stability of silver, zinc oxide, and titanium dioxide nanoparticles and immobilization of Daphnia magna under guideline testing conditions[J]. Ecotoxicology and Environmental Safety, 2016, 127:144-152 Wu B, Zhuang W Q, Sahu M, et al. Cu-doped TiO2 nanoparticles enhance survival of Shewanella oneidensis MR-1 under ultraviolet light (UV) exposure[J]. Science of the Total Environment, 2011, 409(21):4635-4639 Ou Q, Xu Y H, Li X L, et al. Interactions between activated sludge extracellular polymeric substances and model carrier surfaces in WWTPs:A combination of QCM-D, AFM and XDLVO prediction[J]. Chemosphere, 2020, 253:126720 Song J H, Xu Y H, Liu C H, et al. Interpreting the role of NO3-, SO42-, and extracellular polymeric substances on aggregation kinetics of CeO2 nanoparticles:Measurement and modeling[J]. Ecotoxicology and Environmental Safety, 2020, 194:110456 Huangfu X L, Xu Y H, Liu C H, et al. A review on the interactions between engineered nanoparticles with extracellular and intracellular polymeric substances from wastewater treatment aggregates[J]. Chemosphere, 2019, 219:766-783 Khan S S, Mukherjee A, Chandrasekaran N. Impact of exopolysaccharides on the stability of silver nanoparticles in water[J]. Water Research, 2011, 45(16):5184-5190 Xu H C, Jiang H L. Effects of cyanobacterial extracellular polymeric substances on the stability of ZnO nanoparticles in eutrophic shallow lakes[J]. Environmental Pollution, 2015, 197:231-239 Morelli E, Gabellieri E, Bonomini A, et al. TiO2 nanoparticles in seawater:Aggregation and interactions with the green alga Dunaliella tertiolecta[J]. Ecotoxicology and Environmental Safety, 2018, 148:184-193 Yang Y Y, Hou J, Wang P F, et al. Influence of extracellular polymeric substances on cell-NPs heteroaggregation process and toxicity of cerium dioxide NPs to Microcystis aeruginosa[J]. Environmental Pollution, 2018, 242:1206-1216 Davarpanah E, Guilhermino L. Are gold nanoparticles and microplastics mixtures more toxic to the marine microalgae Tetraselmis chuii than the substances individually?[J]. Ecotoxicology and Environmental Safety, 2019, 181:60-68 Chen J Y, Qian Y, Li H R, et al. The reduced bioavailability of copper by nano-TiO2 attenuates the toxicity to Microcystis aeruginosa[J]. Environmental Science and Pollution Research, 2015, 22(16):12407-12414 Dalai S, Pakrashi S, Bhuvaneshwari M, et al. Toxic effect of Cr(Ⅵ) in presence of n-TiO2 and n-Al2O3 particles towards freshwater microalgae[J]. Aquatic Toxicology, 2014, 146:28-37 Iswarya V, Johnson J B, Parashar A, et al. Modulatory effects of Zn2+ ions on the toxicity of citrate-and PVP-capped gold nanoparticles towards freshwater algae, Scenedesmus obliquus[J]. Environmental Science and Pollution Research, 2017, 24(4):3790-3801 -
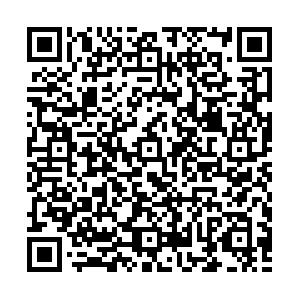
计量
- 文章访问数: 3055
- HTML全文浏览数: 3055
- PDF下载数: 138
- 施引文献: 0