四环素类抗生素耐药研究进展:质粒介导的替加环素耐药机制
Mechanisms of Tetracycline Resistance: Plasmid-Mediated Tigecycline Resistance
-
摘要: 抗生素耐药性已成为全球公共卫生的重大挑战。替加环素作为甘氨酰环素类第三代四环素类抗生素,克服了传统四环素类药物的耐药机制,是治疗产碳青霉烯酶多重耐药细菌感染的“最后一道防线”,被世界卫生组织(WHO)列为极其重要的抗生素。然而,随着质粒介导的高水平替加环素耐药基因的出现,替加环素耐药性呈显著上升趋势。替加环素高水平耐药不仅可导致一代、二代四环素药物失效,也可能对新四环素类抗生素形成交叉耐药,其耐药性的传播扩散将对生态安全和公众健康构成严重威胁。本文详述了四环素类抗生素耐药机制的形成与发展,重点阐述了质粒介导的替加环素耐药基因的产生及其在“动物-环境-人类”中的传播过程,最后基于大数据解析了完整细菌基因组中替加环素耐药基因的多种菌株染色体和质粒分布特征,结果可为遏制替加环素耐药性传播扩散提供科学依据。
-
关键词:
- 四环素 /
- 替加环素 /
- 耐药机制 /
- 质粒 /
- tet(X)基因家族
Abstract: Antibiotic resistance is a major challenge to global public health. As a new type of tetracycline antibiotic, tigecycline overcomes the main tetracycline resistance mechanisms. Therefore, tigecycline is used for the treatment of infections caused by multidrug-resistant Gram-positive and Gram-negative bacteria, and is considered as a last-resort antibiotic against carbapenem-resistant Enterobacteriaceae infections. However, tigecycline resistance showed an increasing trend after the emergence of plasmid-mediated high-level tigecycline resistance genes. Moreover, high-level tigecycline resistance not only leads to the failure of the first and second generation tetracyclines, but also form cross-resistance to other new tetracyclines. The spread of tigecycline resistance genes in animal-environment-human will pose a serious threat to the ecological safety and public health. At present, the mechanisms of tigecycline resistance include active efflux pump transport system, modified enzyme degradation, mutation of ribosomal protein binding site, mutation of cell membrane, etc. Among them, efflux pump and modified enzyme degradation are the main resistance mechanisms. This paper reviews the development of tetracycline resistance mechanisms, emphatically focuses on the plasmid-mediated tigecycline resistance mechanisms. At last, to obtain the prevalence of tetracycline resistance genes in bacterial genomes and plasmids, we analyze a large number of public available bacterial genomic data. This review paper provides scientific references for further monitoring the spread of tigecycline resistance genes.-
Key words:
- tetracycline /
- tigecycline /
- resistance mechanism /
- plasmid /
- tet(X) gene family
-
-
McAllister T A, Wang Y X, Diarra M S, et al. Challenges of a one-health approach to the development of alternatives to antibiotics[J]. Animal Frontiers:The Review Magazine of Animal Agriculture, 2018, 8(2):10-20 Fang L X, Chen C, Cui C Y, et al. Emerging high-level tigecycline resistance:Novel tetracycline destructases spread via the mobile Tet(X)[J]. BioEssays:News and Reviews in Molecular, Cellular and Developmental Biology, 2020, 42(8):e2000014 Michalova E, Novotna P, Schlegelova J. Tetracyclines in veterinary medicine and bacterial resistance to them[J]. Veterinarni Medicina, 2004, 49(3):79-100 Daghrir R, Drogui P. Tetracycline antibiotics in the environment:A review[J]. Environmental Chemistry Letters, 2013, 11(3):209-227 He T, Wang R, Liu D J, et al. Emergence of plasmid-mediated high-level tigecycline resistance genes in animals and humans[J]. Nature Microbiology, 2019, 4(9):1450-1456 Roberts M C, Schwarz S. Tetracycline and phenicol resistance genes and mechanisms:Importance for agriculture, the environment, and humans[J]. Journal of Environmental Quality, 2016, 45(2):576-592 Izaki K, Arima K. Disappearance of oxytetracycline accumulation in the cells of multiple drug-resistant Escherichia coli[J]. Nature, 1963, 200:384-385 Levy S B, McMurry L. Plasmid-determined tetracycline resistance involves new transport systems for tetracycline[J]. Nature, 1978, 276(5683):90-92 Kaneko M, Yamaguchi A, Sawai T. Energetics of tetracycline efflux system encoded by Tn10 in Escherichia coli[J]. FEBS Letters, 1985, 193(2):194-198 Mendez B, Tachibana C, Levy S B. Heterogeneity of tetracycline resistance determinants[J]. Plasmid, 1980, 3(2):99-108 Burdett V. Streptococcal tetracycline resistance mediated at the level of protein synthesis[J]. Journal of Bacteriology, 1986, 165(2):564-569 Burdett V. Purification and characterization of Tet(M), a protein that renders ribosomes resistant to tetracycline[J]. The Journal of Biological Chemistry, 1991, 266(5):2872-2877 Burdett V. Tet(M)-promoted release of tetracycline from ribosomes is GTP dependent[J]. Journal of Bacteriology, 1996, 178(11):3246-3251 Yang W R, Moore I F, Koteva K P, et al. TetX is a flavin-dependent monooxygenase conferring resistance to tetracycline antibiotics[J]. Journal of Biological Chemistry, 2004, 279(50):52346-52352 Grossman T H. Tetracycline antibiotics and resistance[J]. Cold Spring Harbor Perspectives in Medicine, 2016, 6(4):a025387 Zhanel G G, Homenuik K, Nichol K, et al. The glycylcyclines:A comparative review with the tetracyclines[J]. Drugs, 2004, 64(1):63-88 Yaghoubi S, Zekiy A O, Krutova M, et al. Tigecycline antibacterial activity, clinical effectiveness, and mechanisms and epidemiology of resistance:Narrative review[J]. European Journal of Clinical Microbiology & Infectious Diseases, 2022, 41(7):1003-1022 World Health Organization. Critically important antimicrobials for human medicine 2019[R]. Geneva:World Health Organization, 2019 Chen C, Cui C Y, Yu J J, et al. Genetic diversity and characteristics of high-level tigecycline resistance Tet(X) in Acinetobacter species[J]. Genome Medicine, 2020, 12(1):111 Sun J, Chen C, Cui C Y, et al. Plasmid-encoded Tet(X) genes that confer high-level tigecycline resistance in Escherichia coli[J]. Nature Microbiology, 2019, 4(9):1457-1464 Sun C T, Cui M Q, Zhang S, et al. Plasmid-mediated tigecycline-resistant gene Tet(X4) in Escherichia coli from food-producing animals, China, 2008-2018[J]. Emerging Microbes & Infections, 2019, 8(1):1524-1527 Sun C T, Cui M Q, Zhang S, et al. Genomic epidemiology of animal-derived tigecycline-resistant Escherichia coli across China reveals recent endemic plasmid-encoded Tet(X4) gene[J]. Communications Biology, 2020, 3:412 Zhang R M, Sun J, Sun R Y, et al. Source tracking and global distribution of the tigecycline non-susceptible tet(X)[J]. Microbiology Spectrum, 2021, 9(3):e0116421 Livermore D M. Tigecycline:What is it, and where should it be used?[J]. The Journal of Antimicrobial Chemotherapy, 2005, 56(4):611-614 Bradford P A. Tigecycline:A first in class glycylcycline[J]. Clinical Microbiology Newsletter, 2004, 26(21):163-168 Dougherty J A, Sucher A J, Chahine E B, et al. Omadacycline:A new tetracycline antibiotic[J]. The Annals of Pharmacotherapy, 2019, 53(5):486-500 Zhanel G G, Cheung D, Adam H, et al. Review of eravacycline, a novel fluorocycline antibacterial agent[J]. Drugs, 2016, 76(5):567-588 孙广龙, 胡立宏. 四环素类抗生素的研究进展[J]. 药学研究, 2017, 36(1):1-5 Sun G L, Hu L H. Advances in the research of tetracyclines antibiotics[J]. Journal of Pharmaceutical Research, 2017, 36(1):1-5(in Chinese)
Verchère A, Broutin I, Picard M. Photo-induced proton gradients for the in vitro investigation of bacterial efflux pumps[J]. Scientific Reports, 2012, 2:306 Adam Y, Tayer N, Rotem D, et al. The fast release of sticky protons:Kinetics of substrate binding and proton release in a multidrug transporter[J]. Proceedings of the National Academy of Sciences of the United States of America, 2007, 104(46):17989-17994 Zechini B, Versace I. Inhibitors of multidrug resistant efflux systems in bacteria[J]. Recent Patents on Anti-Infective Drug Discovery, 2009, 4(1):37-50 李晶, 付喜爱, 刘耀川, 等. 细菌四环素类药物外排泵的研究新进展[J]. 黑龙江畜牧兽医, 2014(23):48-50 Li J, Fu X A, Liu Y C, et al. New research advances in tetracycline drug efflux pump in bacteria[J]. Heilongjiang Animal Science and Veterinary Medicine, 2014 (23):48-50(in Chinese)
Poole K. Efflux-mediated antimicrobial resistance[J]. The Journal of Antimicrobial Chemotherapy, 2005, 56(1):20-51 Thaker M, Spanogiannopoulos P, Wright G D. The tetracycline resistome[J]. Cellular and Molecular Life Sciences, 2010, 67(3):419-431 Forsberg K J, Patel S, Wencewicz T A, et al. The tetracycline destructases:A novel family of tetracycline-inactivating enzymes[J]. Chemistry & Biology, 2015, 22(7):888-897 McGivern B B, McDonell R K, Morris S K, et al. Novel class 1 integron harboring antibiotic resistance genes in wastewater-derived bacteria as revealed by functional metagenomics[J]. Plasmid, 2021, 114:102563 Zhu Y, Wang C Z, Schwarz S, et al. Identification of a novel tetracycline resistance gene, Tet(63), located on a multiresistance plasmid from Staphylococcus aureus[J]. The Journal of Antimicrobial Chemotherapy, 2021, 76(3):576-581 Somprasong N, Hall C M, Webb J R, et al. Burkholderia ubonensis high-level tetracycline resistance is due to efflux pump synergy involving a novel TetA(64) resistance determinant[J]. Antimicrobial Agents and Chemotherapy, 2021, 65(3):e01767-e01720 Bergeron J, Ammirati M, Danley D, et al. Glycylcyclines bind to the high-affinity tetracycline ribosomal binding site and evade Tet(M)- and Tet(O)-mediated ribosomal protection[J]. Antimicrobial Agents and Chemotherapy, 1996, 40(9):2226-2228 Stein G E, Babinchak T. Tigecycline:An update[J]. Diagnostic Microbiology and Infectious Disease, 2013, 75(4):331-336 Hoban D J, Bouchillon S K, Johnson B M, et al. In vitro activity of tigecycline against 6792 Gram-negative and Gram-positive clinical isolates from the global Tigecycline Evaluation and Surveillance Trial (TEST Program, 2004)[J]. Diagnostic Microbiology and Infectious Disease, 2005, 52(3):215-227 Pournaras S, Koumaki V, Spanakis N, et al. Current perspectives on tigecycline resistance in Enterobacteriaceae:Susceptibility testing issues and mechanisms of resistance[J]. International Journal of Antimicrobial Agents, 2016, 48(1):11-18 Jiang Y S, Yang S S, Deng S L, et al. Epidemiology and resistance mechanisms of tigecycline- and carbapenem-resistant Enterobacter cloacae in Southwest China:A 5-year retrospective study[J]. Journal of Global Antimicrobial Resistance, 2022, 28:161-167 Sun Y, Cai Y, Liu X, et al. The emergence of clinical resistance to tigecycline[J]. International Journal of Antimicrobial Agents, 2013, 41(2):110-116 Villa L, Feudi C, Fortini D, et al. Genomics of KPC-producing Klebsiella pneumoniae sequence type 512 clone highlights the role of RamR and ribosomal S10 protein mutations in conferring tigecycline resistance[J]. Antimicrobial Agents and Chemotherapy, 2014, 58(3):1707-1712 Beabout K, Hammerstrom T G, Perez A M, et al. The ribosomal S10 protein is a general target for decreased tigecycline susceptibility[J]. Antimicrobial Agents and Chemotherapy, 2015, 59(9):5561-5566 Cattoir V, Isnard C, Cosquer T, et al. Genomic analysis of reduced susceptibility to tigecycline in Enterococcus faecium[J]. Antimicrobial Agents and Chemotherapy, 2015, 59(1):239-244 Li X, Liu L, Ji J, et al. Tigecycline resistance in Acinetobacter baumannii mediated by frameshift mutation in plsC, encoding 1-acyl-sn-glycerol-3-phosphate acyltransferase[J]. European Journal of Clinical Microbiology & Infectious Diseases, 2015, 34(3):625-631 Li X, Quan J, Yang Y, et al. Abrp, a new gene, confers reduced susceptibility to tetracycline, glycylcine, chloramphenicol and fosfomycin classes in Acinetobacter baumannii[J]. European Journal of Clinical Microbiology & Infectious Diseases:Official Publication of the European Society of Clinical Microbiology, 2016, 35(8):1371-1375 Yu R, Zhang Y, Xu Y D, et al. Emergence of a tet(M) variant conferring resistance to tigecycline in Streptococcus suis[J]. Frontiers in Veterinary Science, 2021, 8:709327 Webber M A, Piddock L J. Absence of mutations in marRAB or soxRS in acrB-overexpressing fluoroquinolone-resistant clinical and veterinary isolates of Escherichia coli[J]. Antimicrobial Agents and Chemotherapy, 2001, 45(5):1550-1552 Hornsey M, Ellington M J, Doumith M, et al. Tigecycline resistance in Serratia marcescens associated with up-regulation of the SdeXY-HasF efflux system also active against ciprofloxacin and cefpirome[J]. The Journal of Antimicrobial Chemotherapy, 2010, 65(3):479-482 Veleba M, De Majumdar S, Hornsey M, et al. Genetic characterization of tigecycline resistance in clinical isolates of Enterobacter cloacae and Enterobacter aerogenes[J]. The Journal of Antimicrobial Chemotherapy, 2013, 68(5):1011-1018 Lv L C, Wan M, Wang C Z, et al. Emergence of a plasmid-encoded resistance-nodulation-division efflux pump conferring resistance to multiple drugs, including tigecycline, in Klebsiella pneumoniae[J]. mBio, 2020, 11(2):e02930-e02919 Pournaras S, Koumaki V, Gennimata V, et al. In vitro activity of tigecycline against Acinetobacter baumannii:Global epidemiology and resistance mechanisms[J]. Advances in Experimental Medicine and Biology, 2016, 897:1-14 Keeney D, Ruzin A, McAleese F, et al. MarA-mediated overexpression of the AcrAB efflux pump results in decreased susceptibility to tigecycline in Escherichia coli[J]. The Journal of Antimicrobial Chemotherapy, 2008, 61(1):46-53 He F, Fu Y, Chen Q, et al. Tigecycline susceptibility and the role of efflux pumps in tigecycline resistance in KPC-producing Klebsiella pneumoniae[J]. PLoS One, 2015, 10(3):e0119064 Veleba M, Higgins P G, Gonzalez G, et al. Characterization of RarA, a novel AraC family multidrug resistance regulator in Klebsiella pneumoniae[J]. Antimicrobial Agents and Chemotherapy, 2012, 56(8):4450-4458 Jiménez-Castellanos J C, Wan Ahmad Kamil W N, Cheung C H P, et al. Comparative effects of overproducing the AraC-type transcriptional regulators MarA, SoxS, RarA and RamA on antimicrobial drug susceptibility in Klebsiella pneumoniae[J]. The Journal of Antimicrobial Chemotherapy, 2016, 71(7):1820-1825 Chen J, Kuroda T, Huda M N, et al. An RND-type multidrug efflux pump SdeXY from Serratia marcescens[J]. The Journal of Antimicrobial Chemotherapy, 2003, 52(2):176-179 Kumar A, Worobec E A. HasF, a TolC-homolog of Serratia marcescens, is involved in energy-dependent efflux[J]. Canadian Journal of Microbiology, 2005, 51(6):497-500 Li R C, Peng K, Xiao X, et al. Emergence of a multidrug resistance efflux pump with carbapenem resistance gene blaVIM-2 in a Pseudomonas putida megaplasmid of migratory bird origin[J]. The Journal of Antimicrobial Chemotherapy, 2021, 76(6):1455-1458 He R W, Yang Y Q, Wu Y P, et al. Characterization of a plasmid-encoded resistance-nodulation-division efflux pump in Klebsiella pneumoniae and Klebsiella quasipneumoniae from patients in China[J]. Antimicrobial Agents and Chemotherapy, 2021, 65(2):e02075-e02020 Hirabayashi A, Ha V, Nguyen A V, et al. Emergence of a plasmid-borne tigecycline resistance in Klebsiella pneumoniae in Vietnam[J]. Journal of Medical Microbiology, 2021, 70(3):10.1099/jmm.0.001320 Wan M, Gao X, Lv L C, et al. IS 26 mediates the acquisition of tigecycline resistance gene cluster tmexCD1-toprJ1 by IncHI1B-FIB plasmids in Klebsiella pneumoniae and Klebsiella quasipneumoniae from food market sewage[J]. Antimicrobial Agents and Chemotherapy, 2021, 65(3):e02178-e02120 Wang C Z, Gao X, Yang Q W, et al. A novel transferable resistance-nodulation-division pump gene cluster, tmexCD2-toprJ2, confers tigecycline resistance in Raoultella ornithinolytica[J]. Antimicrobial Agents and Chemotherapy, 2021, 65(4):e02229-e02220 Wang Q, Peng K, Liu Y, et al. Characterization of TMexCD3-TOprJ3, an RND-type efflux system conferring resistance to tigecycline in Proteus mirabilis, and its associated integrative conjugative element[J]. Antimicrobial Agents and Chemotherapy, 2021, 65(7):e0271220 Hirabayashi A, Dao T D, Takemura T, et al. A transferable IncC-IncX3 hybrid plasmid cocarrying blaNDM-4, tet(X), and tmexCD3-toprJ3 confers resistance to carbapenem and tigecycline[J]. mSphere, 2021, 6(4):e0059221 Peleg A Y, Adams J, Paterson D L. Tigecycline efflux as a mechanism for nonsusceptibility in Acinetobacter baumannii[J]. Antimicrobial Agents and Chemotherapy, 2007, 51(6):2065-2069 Lucaßen K, Müller C, Wille J, et al. Prevalence of RND efflux pump regulator variants associated with tigecycline resistance in carbapenem-resistant Acinetobacter baumannii from a worldwide survey[J]. The Journal of Antimicrobial Chemotherapy, 2021, 76(7):1724-1730 Yang Y S, Chen H Y, Hsu W J, et al. Overexpression of AdeABC efflux pump associated with tigecycline resistance in clinical Acinetobacter nosocomialis isolates[J]. Clinical Microbiology and Infection, 2019, 25(4):512.e1-512.e6 Sengeløv G, Halling-Sørensen B, Aarestrup F M. Susceptibility of Escherichia coli and Enterococcus faecium isolated from pigs and broiler chickens to tetracycline degradation products and distribution of tetracycline resistance determinants in E. coli from food animals[J]. Veterinary Microbiology, 2003, 95(1-2):91-101 Møller T S B, Overgaard M, Nielsen S S, et al. Relation between tetR and tetA expression in tetracycline resistant Escherichia coli[J]. BMC Microbiology, 2016, 16:39 Hentschke M, Christner M, Sobottka I, et al. Combined ramR mutation and presence of a Tn1721-associated Tet(A) variant in a clinical isolate of Salmonella enterica serovar Hadar resistant to tigecycline[J]. Antimicrobial Agents and Chemotherapy, 2010, 54(3):1319-1322 Xu J, Zhu Z L, Chen Y M, et al. The plasmid-borne tet(A) gene is an important factor causing tigecycline resistance in ST11 carbapenem-resistant Klebsiella pneumoniae under selective pressure[J]. Frontiers in Microbiology, 2021, 12:644949 Chiu S K, Huang L Y, Chen H, et al. Roles of ramR and tet(A) mutations in conferring tigecycline resistance in carbapenem-resistant Klebsiella pneumoniae clinical isolates[J]. Antimicrobial Agents and Chemotherapy, 2017, 61(8):e00391-e00317 De Jesus M, Jin J, Guffanti A A, et al. Importance of the GP dipeptide of the antiporter motif and other membrane-embedded proline and glycine residues in tetracycline efflux protein Tet(L)[J]. Biochemistry, 2005, 44(38):12896-12904 Ginn S L, Brown M H, Skurray R A. Membrane topology of the metal-tetracycline/H+ antiporter TetA(K) from Staphylococcus aureus[J]. Journal of Bacteriology, 1997, 179(11):3786-3789 Jin J, Guffanti A A, Bechhofer D H, et al. Tet(L) and Tet(K) tetracycline-divalent metal/H+ antiporters:Characterization of multiple catalytic modes and a mutagenesis approach to differences in their efflux substrate and coupling ion preferences[J]. Journal of Bacteriology, 2002, 184(17):4722-4732 Schwarz S, Cardoso M, Wegener H C. Nucleotide sequence and phylogeny of the Tet(L) tetracycline resistance determinant encoded by plasmid pSTE1 from Staphylococcus hyicus[J]. Antimicrobial Agents and Chemotherapy, 1992, 36(3):580-588 Jiang N S, Li J, Feßler A T, et al. A novel small Tet(T)-Tet(L)-aadD-carrying plasmid from MRSA and MSSA ST9 isolates of swine origin[J]. The Journal of Antimicrobial Chemotherapy, 2019, 74(8):2462-2464 Chopra I, Roberts M. Tetracycline antibiotics:Mode of action, applications, molecular biology, and epidemiology of bacterial resistance[J]. Microbiology and Molecular Biology Reviews, 2001, 65(2):232-260 Kehrenberg C, Catry B, Haesebrouck F, et al. Tet(L)-mediated tetracycline resistance in bovine Mannheimia and Pasteurella isolates[J]. The Journal of Antimicrobial Chemotherapy, 2005, 56(2):403-406 Fiedler S, Bender J K, Klare I, et al. Tigecycline resistance in clinical isolates of Enterococcus faecium is mediated by an upregulation of plasmid-encoded tetracycline determinants Tet(L) and Tet(M)[J]. The Journal of Antimicrobial Chemotherapy, 2016, 71(4):871-881 Wang N N, Li D X, Schwarz S, et al. Novel Tet(L) efflux pump variants conferring resistance to tigecycline and eravacycline in Staphylococcus spp.[J]. Microbiology Spectrum, 2021, 9(3):e0131021 Yao H, Jiao D, Zhao W B, et al. Emergence of a novel tet(L) variant in Campylobacter spp. of chicken origin in China[J]. Antimicrobial Agents and Chemotherapy, 2020, 65(1):e01622-e01620 Tally F P, Snydman D R, Shimell M J, et al. Characterization of pBFTM10, a clindamycin-erythromycin resistance transfer factor from Bacteroides fragilis[J]. Journal of Bacteriology, 1982, 151(2):686-691 Guiney D G Jr, Hasegawa P, Davis C E. Expression in Escherichia coli of cryptic tetracycline resistance genes from Bacteroides R plasmids[J]. Plasmid, 1984, 11(3):248-252 Matthews B G, Guiney D G. Characterization and mapping of regions encoding clindamycin resistance, tetracycline resistance, and a replication function on the Bacteroides R plasmid pCP1[J]. Journal of Bacteriology, 1986, 167(2):517-521 Speer B S, Salyers A A. Novel aerobic tetracycline resistance gene that chemically modifies tetracycline[J]. Journal of Bacteriology, 1989, 171(1):148-153 Speer B S, Salyers A A. A tetracycline efflux gene on Bacteroides transposon Tn4400 does not contribute to tetracycline resistance[J]. Journal of Bacteriology, 1990, 172(1):292-298 Whittle G, Hund B D, Shoemaker N B, et al. Characterization of the 13-kilobase ermF region of the Bacteroides conjugative transposon CTnDOT[J]. Applied and Environmental Microbiology, 2001, 67(8):3488-3495 Li R C, Liu Z Y, Peng K, et al. Co-occurrence of two tet(X) variants in an Empedobacter brevis of shrimp origin[J]. Antimicrobial Agents and Chemotherapy, 2019, 63(12):e01636-e01619 Dong N, Zeng Y, Cai C, et al. Prevalence, transmission, and molecular epidemiology of Tet(X)-positive bacteria among humans, animals, and environmental niches in China:An epidemiological, and genomic-based study[J]. The Science of the Total Environment, 2022, 818:151767 Li R C, Lu X Y, Peng K, et al. Deciphering the structural diversity and classification of the mobile tigecycline resistance gene tet(X)-bearing plasmidome among bacteria[J]. mSystems, 2020, 5(2):e00134-e00120 Wang L Y, Liu D J, Lv Y, et al. Novel plasmid-mediated tet(X5) gene conferring resistance to tigecycline, eravacycline, and omadacycline in a clinical Acinetobacter baumannii isolate[J]. Antimicrobial Agents and Chemotherapy, 2019, 64(1):e01326-e01319 He D D, Wang L L, Zhao S Y, et al. A novel tigecycline resistance gene, Tet(X6), on an SXT/R391 integrative and conjugative element in a Proteus genomospecies 6 isolate of retail meat origin[J]. The Journal of Antimicrobial Chemotherapy, 2020, 75(5):1159-1164 Gasparrini A J, Markley J L, Kumar H, et al. Tetracycline-inactivating enzymes from environmental, human commensal, and pathogenic bacteria cause broad-spectrum tetracycline resistance[J]. Communications Biology, 2020, 3:241 Soliman A M, Ramadan H, Zarad H, et al. Coproduction of Tet(X7) conferring high-level tigecycline resistance, fosfomycin FosA4, and colistin mcr-1.1 in Escherichia coli strains from chickens in Egypt[J]. Antimicrobial Agents and Chemotherapy, 2021, 65(6):e02084-e02020 Cheng Y Y, Chen Y, Liu Y, et al. Identification of novel tetracycline resistance gene Tet(X14) and its co-occurrence with Tet(X2) in a tigecycline-resistant and colistin-resistant Empedobacter stercoris[J]. Emerging Microbes & Infections, 2020, 9(1):1843-1852 Liu D J, Zhai W S, Song H W, et al. Identification of the novel tigecycline resistance gene Tet(X6) and its variants in Myroides, Acinetobacter and Proteus of food animal origin[J]. The Journal of Antimicrobial Chemotherapy, 2020, 75(6):1428-1431 Xu Y C, Liu L Z, Zhang H M, et al. Co-production of Tet(X) and MCR-1, two resistance enzymes by a single plasmid[J]. Environmental Microbiology, 2021, 23(12):7445-7464 Umar Z, Chen Q W, Tang B, et al. The poultry pathogen Riemerella anatipestifer appears as a reservoir for Tet(X) tigecycline resistance[J]. Environmental Microbiology, 2021, 23(12):7465-7482 Cui C Y, Chen Q W, He Q, et al. Transferability of tigecycline resistance:Characterization of the expanding Tet(X) family[J]. WIREs Mechanisms of Disease, 2022, 14(1):e1538 王知任, 李荷楠. 质粒介导的替加环素耐药机制的研究进展[J]. 中国感染与化疗杂志, 2021, 21(2):230-234 Wang Z R, Li H N. Recent advances in plasmid-mediated mechanism of tigecycline resistance[J]. Chinese Journal of Infection and Chemotherapy, 2021, 21(2):230-234(in Chinese)
王亮亮, 王倩倩, 周博伦, 等. 替加环素耐药机制研究进展[J]. 中国畜牧兽医, 2020, 47(9):3022-3029 Wang L L, Wang Q Q, Zhou B L, et al. Advance on the tigecycline resistance mechanism[J]. China Animal Husbandry & Veterinary Medicine, 2020, 47(9):3022-3029(in Chinese)
Parks D H, Chuvochina M, Waite D W, et al. A standardized bacterial taxonomy based on genome phylogeny substantially revises the tree of life[J]. Nature Biotechnology, 2018, 36(10):996-1004 Alcock B P, Raphenya A R, Lau T T Y, et al. CARD 2020:Antibiotic resistome surveillance with the comprehensive antibiotic resistance database[J]. Nucleic Acids Research, 2020, 48(D1):D517-D525 Hu S H, Jiang T, Zhou Y J, et al. Genomic analysis of the multi-drug-resistant clinical isolate Myroides odoratimimus PR63039[J]. Molecular Genetics and Genomics, 2017, 292(1):133-144 -
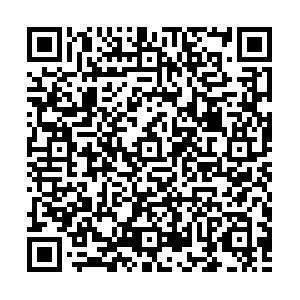
计量
- 文章访问数: 5894
- HTML全文浏览数: 5894
- PDF下载数: 240
- 施引文献: 0